NOTIFICATIONS
Antibacterial effects of honey – experiment.
- + Create new collection
Honey is antibacterial and can prevent growth of most types of bacteria. This experiment compares the effect of different types of honey on bacteria growing on agar plates.
To compare the antibacterial effect of different honey types.
Honey, antibacterial, bacteria, agar, experiment.
See our newsletters here .

Would you like to take a short survey?
This survey will open in a new tab and you can fill it out after your visit to the site.
An official website of the United States government
Official websites use .gov A .gov website belongs to an official government organization in the United States.
Secure .gov websites use HTTPS A lock ( Lock Locked padlock icon ) or https:// means you've safely connected to the .gov website. Share sensitive information only on official, secure websites.
- Publications
- Account settings
- Advanced Search
- Journal List
The Antibacterial Activity of Honey on Helicobacter Pylori
Basil c nzeako, faiza al-namaani.
- Author information
- Copyright and License information
To whom correspondence should be addressed. Email: [email protected]
This project aimed to assess the antibacterial potential of various brands of honey sold in Muscat area on some isolates of H. pylori and to determine if there is any synergy between honey and amoxycillin or clarithromycin used in the treatment of H. pylori gastritis and duodenal ulcer.
Eight samples of commercial honey were used in the experiment after they were checked for purity by sub-culturing on blood agar and incubating for 48 hours at 37°c. Honey samples showing gross contamination were discarded. Purified culture isolates of H. pylori from our laboratory stock cultures were swabbed on chocolate plate using 1x 10 4 cfu/ml. One hundred microlitres (100μl) of various honey samples were placed on each plate which was subsequently incubated microaerophilically at 37ºc for 3 days. The presence or absence of growth inhibition zones on each plate was noted and an average zone size of each honey was taken. Honey samples with high zone sizes were further diluted from 1:2–1:8 to find the end-points of their growth inhibition concentrations and the experiment was repeated in triplicates. The synergistic effect between honey, amoxycillin and clarithromycin was done in triplicates by placing honey at various distances between each antibiotic after swabbing chocolate agar with 1x 10 4 cfu/ml of H. pylori. The plates were incubated as before.
All honey samples produced growth inhibition zones with H. pylori no at dilution of honey but had different zone sizes at 1:2–1:8 dilutions. Black Forest honey had the highest antibacterial activity followed by Langnese honey. None of the honeys had a synergistic effect with either clarithromycin or amoxycillin.
Conclusion:
We conclude that, in vitro, some honey brands possess antibacterial activity against H. pylori and that no synergy or antagonism was observed between honey and clarithromycin or honey and amoxicillin using H. pylori as a test organism. Though no synergy or antagonism was observed between honey, amoxicillin or clarithromycin, it has been suggested that the use of honey with triple therapy regimen may help shorten the time required to eliminate H. pylori from stomach lining of patients with gastritis or duodenal ulcer caused by H. pylori infection
Keywords: Antibacterial, Amoxicillin, Clarithromycin, Honey, H. pylori , Triple therapy, Synergy
T he history of H elicobacter pylori dates back to 1875 when the German scientists first found spiral bacteria in the lining of the human stomach. 1 Walery in 1899 published a paper on spiral shaped bacteria from the sediment of human gastric washings, but his publication was in Polish so it attracted little or no attention. 2
The work of the German and Polish scientists was not rediscovered till 1979 when Warren saw curved and spiral bacteria in the biopsy material from gastric mucosa. He and Marshall isolated and cultured the organism and named it Campylobacter pyloridis . 3 They contended that most stomach ulcers and gastritis were caused by colonization with this bacterium. Following DNA sequences, the organism in 1989 was renamed Helicobacter pylori by Warren and Marshall. 4
However, the association of H. pylori with gastritis or ulcers was distrusted since it was believed that no bacterium could survive for long in the acid pH of the stomach. To prove their point, Marshall infected himself and had gastritis. The organism was subsequently isolated from his stomach lining. 5 Marshall and Warren were awarded the Nobel Prize in medicine in 2005 for their discovery of H. pylori and its role in gastritis and peptic ulcer disease. 6
Currently, it is estimated that half the world’s population harbours H. pylori and that while it exits as normal flora in some people, in others, it causes gastritis and ulcers. 7
Malfertheiner et al. 8 recommended the use of triple therapy which includes an anti-ulcer agent such as ranitidine, bismuth citrate, or a proton pump inhibitor, omeprazol or pantoprazole and a combination of two antibiotics from either metronidazole, clarithromycin or amoxicillin for elimination of H. pylori from the stomach. Though this recommendation was made and is applied in the treatment of H. pylori infection, a significant problem associated with the treatment is the organism’s increasing resistance to antibiotics and the failure of the clinicians to follow up the course of treatment with repeated isolations and sensitivity pattern of the original isolate. Hiyana 9 in Japan found most of his H. pylori isolates to be resistant to clarithromycin and metronidazole while Crone et al. 10 isolates from children and adolescent, in vitro , showed resistance to clarithromycin.
Honey has been used as a medicine since ancient times in many countries. 11 , 12 , 13 Its use is recorded in Sumerian clay tablets estimated to be 4000 years old and in Egyptian papyri from 1900 to 1250 B.C. 11 The revelation in the Holy Koran and documentation in the Hadith clearly referred to the effectiveness of honey in the healing of diseases for mankind. 14 Abu Taib et al. 14 reported that growth of H. pylori was inhibited by 20% concentration of natural honey while Al Somal et al, 15 Mcgovern et al. 16 found, in vitro, that manuka honey solution possessed antibacterial properties against H. pylori .
Our project aimed to determine the antibacterial potential of various brands of honey sold in Muscat area on H. pylori and to find out if there is synergy between honey and amoxycillin or clarithromycin which are two antibiotics currently in use in the treatment of H. pylori infection in Sultan Qaboos University Hospital (SQUH).
CULTURES OF HELICOBACTER PYLORI
These were provided from departmental stock cultures. The organisms were checked for purity by inoculating them onto Campylobacter and chocolate agar media and incubating microaerophilically (CO2 10%, Oxygen 10%, Nitrogen 80%) for 5 days at 37°C. Grown colonies were Gram stained and observed for spiral shape, oxidase and urease activities. Spiral shaped, urease and oxidase positive colonies typical of H. pylori were used in the experiment.
HONEY SAMPLES
Thirteen brands of commercial honey available in Muscat area of Oman were collected for the study. They were checked for purity by inoculating onto blood agar and incubating aerobically at 37°C for 48 hours. Samples showing growth of bacteria or growth of more than 4–5 colonies of yeasts were excluded from the study. Only eight samples were found good for our study [ Table 1 ].
Honey samples and their sources
CHECKING THE HONEY SAMPLES FOR ANTIBACTERIAL ACTIVITY
The honeys were initially checked for antibacterial activity by using Staphylococcus aureus (NCTC 6571) as a control organism. This strain of S. aureus is known to be sensitive to most antimicrobial agents and is used in our laboratory for the control of our antibiotic sensitivity pattern. An inoculum containing 1x10 4 cfu/ml of the organism was prepared using McFarland tube 0.5. A dry swab immersed into it was pressed on the side of the bottle to remove excess fluid and was subsequently used to swab a dry diagnostic sensitivity test (DST, Oxoid, UK) plate. One hundred microlitres (100μl) of honey was placed on the surface of each plate using a chipped sterile tip. This process was applied for each honey sample. The plates were incubated at 37°C overnight and zones of growth inhibition produced by each honey with the test organism were subsequently taken.
DETERMINATION OF ANTIBACTERIAL ACTIVITY OF HONEY ON H. PYLORI
Inocula containing 1x 10 4 cfu/ml of each H. pylori were prepared and smeared on chocolate as done with S. aureus control. One hundred microliters (100μl) of each individual honey were placed on the surface of each plate using a chipped sterile tip as before. The plates were incubated microerophilically (CO2 10%, Oxygen 10%, Nitrogen 80%) at 37°C for 3 days. Honey samples producing zones of inhibition of more than 8mm were double diluted from 1:2 to 1:8 to obtain the final end-points of antibacterial activity.
SYNERGISTIC TEST BETWEEN HONEY AND SOME ANTIBIOTICS
The antibiotics used in this experiment were those used for the treatment of cases of H. pylori infection at SQUH. Amoxycillin (Panpharma, France) and clarithromycin (Panpharma, France) obtained from the Pharmacy Department of the SQUH at concentrations of 250mg powder were diluted to 10μg and 15μg respectively using sterile distilled water. These concentrations are contained in the antibiotic discs routinely used at Sultan Qaboos University Hospital for the determination of H. pylori sensitivity. Efforts to obtain the antibiotics as pure liquid concentrates proved impossible.
Two isolates of H. pylori out of five used in this experiment were employed. An inoculum of each H. pylori was prepared and smeared on plates as mentioned earlier in the text. Fifty microlitres (50μl) of honey and antibiotics were placed at 15, 20 and 25mm apart from each other using three sets of plates per distance and for each antibiotic. They were allowed to seep into the media and incubated microaerophilically (CO2 10%, Oxygen 10%, Nitrogen 80%) at 37ºC for 3 days. Synergy exists if the growth inhibition zone produced by honey in combination with any of the antibiotics is greater than growth inhibition zones produced by either alone. 17
Table 1 shows the types of honey and their sources while figure 1 is a micrograph of the growth inhibition zones produced by some honey on S. aureus (control organism). Table 2 shows the inhibition zone sizes produced by various honeys at various dilutions of the honeys. The highest growth inhibition zone was produced by Black Forest (sample 1) followed by Langnese honey and natural honeys (samples 2 and 3). Other honeys used in the investigation produced zones only at no dilution. Figure 2 represents the micrograph of the growth inhibition zones produced by some of the honey samples on H. pylori on chocolate agar.
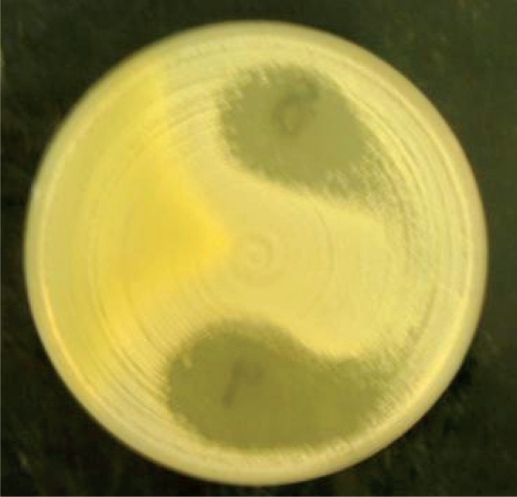
Zone of inhibition of honey on Staphylococcus aureus (NCTC6571).
The zone of growth inhibition of the honey samples on H. pylori
Not done (insufficient amount of honey)
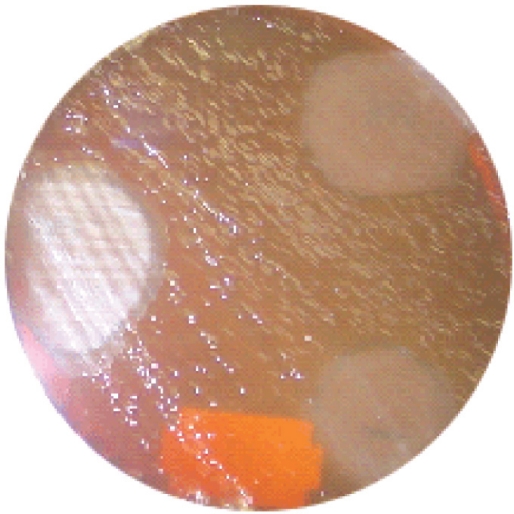
Zone of inhibition of honey on Helicobacter pylori on chocolate agar.
No synergy was observed between honey and clarithromycin or honey and amoxicillin.
Honey is known to possess antimicrobial activity and has been used to cure many skin infections. 11 , 12 , 13 , 18 , 19 In our experiment, we observed that all the honey samples examined possessed antibacterial activity against H. pylori with the natural Black Forest honey from the Black Forest in Germany showing the highest antibacterial activity. This is followed by Langnese honey also from Germany.
The elements responsible for the antibacterial activity of honey are not fully known, but have been attributed to various polyphenolic compounds found in honey (propolis, flavonoids, flavones, tannins) and to glucose oxidase and osmosis. 11 Honey is a product of various plant nectars and differences exist between one nectar and the other based on plant species, the season of the year and the geographical location of the plant. 11 Molan 11 , 19 attributed the antibacterial activity of honey to its content of glucose oxidase, which liberates hydrogen peroxide when it is diluted. The hydrogen peroxide so liberated is antibacterial besides encouraging epithelial proliferation.
Burdon 20 emphasized that the normal route of wound healing is the stimulation of the growth of fibroblasts and epithelial cells by the hydrogen peroxide produced as a result of injury or infection. Somerfield 21 , Condon 22 and Osato 23 ascribed the antibacterial effect of honey to the osmotic effect of its sugar content. Honey contains 38% fructose, 31% glucose and 17% water while its pH is 3.9. 24 Its low content of water encourages wound healing by hygroscopic absorption of water molecules on wound surfaces and by soothing of the wound. 12 , 13
The findings of our work agree with the observation of many earlier researchers 14 , 15 , 16 , 19 , 23 , 25 who, in vitro, found honey to possess antibacterial activity against H. pylori .
In SQUH, amoxicillin and clarithromycin are used in the treatment of Helicobacter infection using triple regimen. 26 The use of triple regimen is based on the fact that antibiotics will eliminate H. pylori allowing gastric or duodenal ulcers to heal naturally. 8
This condition is not always obtained for some patients fail to comply with the treatment regimen, thereby creating a lack of sufficient concentration of antibiotics in the stomach over a period required to eliminate the organism. In addition, there is an increase in gastric pH which affects the activity of some of the drugs. The low content of the drugs in the stomach results in bacterial mutation, increase of bacterial load and subsequent emergence of intracellular and dormant forms of the bacterium which renders the drugs ineffective. 27
Though in our experiment, we found no synergy existing between amoxicillin or clarithromycin and honey, suggestions were made that the combination of triple regimen with any of the agents known, in vitro, to have antibacterial effect against H. pylori could help eliminate the organism from the stomach. 19 , 27 In this respect, suggestions were advanced of combining the triple therapy regimen with fresh or fermented milk, propolis, broccoli sprouts, ginger, honey, or mangava-brava. 27 , 28 , 29 , 30 , 31 , 32 , 33 Fresh milk contains lactoferrin, fermented milk (lactoferrin and lactobacilli), propolis (polyphenols), broccoli sprouts (sulforaphane) ginger (gingerols), honey (glucose oxidase and polyphenols), mangava-brava (ellagic acid). These substances, individually, are antibacterial and are known in vitro , to stop the growth of H. pylori , but whether, in combination with the triple therapy, more effective elimination of H. pylori is ensured is subject to further experiment, though synergy has been shown to exists between honey and some antibiotics. 34
However, the advantage which honey has over other substances suggested is that honey has a pH of 3.9. 24 and its dilution by gastric juices in the stomach will activate its glucose oxidase content. The glucose oxidase so activated will liberate hydrogen peroxide which is both antibacterial and an activator of fibroblasts and epithelial cells needed in the healing process of the ulcer caused by H. pylori. Helicobacter pylori thrives in acid pH, but produces infection under alkaline pH due to its ability to hydrolyze urea from mucosa cells liberating ammonia 27 . The acid pH of honey can neutralize this thereby helping the healing process of ulcer caused by the organism. The drawback is that it is difficult to quantify the dosage, but it is worth experimenting on.
In our experiment, we found the use of the surface diffusion method better than well diffusion. In surface diffusion, contaminants in honey had more surface area for growth and were clearly visible as contaminants but this was absent in the well diffusion method. Moreover, viscous honey samples diffused poorly in the well diffusion method resulting in little or no growth inhibition zones being produced.
In summary, we have tried to show that in vitro many honey samples sold in Muscat area of Oman possess antibacterial activity against H. pylori and that the degree of this antibacterial activity varies from sample to sample. The differences in antibacterial activity, in our opinion, are dependent on where the nectars were obtained by the bees, the mode of preparation and preservation of the honeys and how long the honeys stayed on the shelves before use. We found that no synergy existed between honey and amoxicillin or clarithromycin used in the treatment of H. pylori infection. Though no synergy was observed between honey and the antibiotics used in our experiment, we suggest that honey used in combination with triple therapy may help speed up the rate of elimination of H. pylori from cases of gastritis and duodenal ulcer patients.
Acknowledgments
The authors are most grateful to Dr. Akbar Rafay for providing all the stock cultures of H. pylori used in the experiment, the Pharmacy Department of SQUH for providing the antibiotics for synergy experiment and to the staff of Microbiology and Immunology for their cooperation through out the period of the investigation.
- 1. Blasser MJ. An endangered species in the stomach. Sci Am. 2005;292:38–45. doi: 10.1038/scientificamerican0205-38. [ DOI ] [ PubMed ] [ Google Scholar ]
- 2. Konturek JW. Discovery by Jaworski of Helicobacter pylori and its pathogenic role in peptic ulcer, gastritis and gastric cancer. J Physiol Pharmacol. 2003;54:23–41. [ PubMed ] [ Google Scholar ]
- 3. Marshall BJ. Unidentified curved bacilli on gastric epithelium in active chronic gastritis. Lancet. 1983;1:1273–1275. [ PubMed ] [ Google Scholar ]
- 4. Marshall BJ, Warren JR. Unidentified curved bacilli in the stomach patients with gastritis and peptic ulceration. Lancet. 1984;1:1311–1315. doi: 10.1016/s0140-6736(84)91816-6. [ DOI ] [ PubMed ] [ Google Scholar ]
- 5. Helicobacter pylori in peptic ulcer disease NIH Consensus Statement online Jan 7, 1994; 12:1023. [ PubMed ] [ Google Scholar ]
- 6. The Nobel Prize in Physiology or Medicine awarded to Marshall BJ and Warren JR “For their discovery of the bacterium Helicobacter pylori and its role in gastritis and peptic ulcer disease”. Nobelprize.org/med/laureates/2005/index.html, Accessed 9 th September 2006.
- 7. Angela CM, Charles WP. Helicobacter pylori. In: Sussman Max., editor. Molecular Medical Microbiology. London: Academic Press; 2002. pp. 1331–1347. [ Google Scholar ]
- 8. Malfertheiner P, Megraud F, O’Morain C, et al. Current concepts in the management of Helicobacter pylori infection: The Maastricht 2–2000 Consensus Report. Aliment Pharmacol Ther. 2002;16:167–80. doi: 10.1046/j.1365-2036.2002.01169.x. [ DOI ] [ PubMed ] [ Google Scholar ]
- 9. Hiyana T, Tanaka S, Masuda H, et al. Prevalence of Helicobacter pylori resistance to clarithromycin and metronidazole determined by 23s ribosomal RNA and rdxA gene analyses in Hiroshima, Japan. J Gastroenterol Hepatol. 2003;18:1202–1207. doi: 10.1046/j.1440-1746.2003.03140.x. [ DOI ] [ PubMed ] [ Google Scholar ]
- 10. Crone J, Granditsch G, Huber WD, et al. Helicobacter pylori in children and adolescent: Increase of primary clarithromycin resistance 1997–2000. J Paediatr Gastreenterol Nutr. 2003;36:368–371. doi: 10.1097/00005176-200303000-00012. [ DOI ] [ PubMed ] [ Google Scholar ]
- 11. Molan PC. The antibacterial activity of honey: 1 The nature of the antibacterial activity. J Bee World. 1992;73:5–28. [ Google Scholar ]
- 12. Efem SE. Recent advances in the management of Fournier’s gangrene: Preliminary observations. J Surg. 1993;113:200–204. [ PubMed ] [ Google Scholar ]
- 13. Efem SE. Clinical observation on the wound healing properties of honey. Br J Surg. 1988;75:679–681. doi: 10.1002/bjs.1800750718. [ DOI ] [ PubMed ] [ Google Scholar ]
- 14. Abu Taib MM, Chowdhury MN, Al-Humayyd M. Inhibitory effect of natural honey on Helicobacter pylori. Trop Gastroenterol. 1991;12:139–143. [ PubMed ] [ Google Scholar ]
- 15. Al-Somal N, Coley KE, Molan PC, Hancock BM. Susceptibility of Helicobacter pylori to the antibacterial activity of manuka honey. J Royal Soc Med. 1994;87:9–13. doi: 10.1177/014107689408700106. [ DOI ] [ PMC free article ] [ PubMed ] [ Google Scholar ]
- 16. Mc-Govern PB, Abbas SZ, Vivian G, Dalton HR. Manuka honey against Helicobacter pylori. J Royal Soc Med. 1999;92:439. doi: 10.1177/014107689909200832. [ DOI ] [ PMC free article ] [ PubMed ] [ Google Scholar ]
- 17. Miles RS, Amyes SGB. Laboratory control of antimicrobial therapy. In: Collee JG, Fraser AG, Marmion BP, Simmons A, editors. Mackie and McCartney, Practical Medical Microbiology. 14th Ed. Edinburgh: Churchill Livingstone; 1996. p. 175. [ Google Scholar ]
- 18. Dunford C, Cooper RA, Molan PC. Using honey as a dressing for infected skin lesions. Nurs. 2000;96:7–9. [ PubMed ] [ Google Scholar ]
- 19. Molan PC. Why honey is effective as a medicine:The scientific explanation of its effects. Bee World. 2001;82:22–40. [ Google Scholar ]
- 20. Burdon RH. Superoxide and hydrogen peroxide in relation to mammalian cell proliferation. Free Radic Biol Med. 1995;18:775–794. doi: 10.1016/0891-5849(94)00198-s. [ DOI ] [ PubMed ] [ Google Scholar ]
- 21. Somerfield SD. Honey and healing. J R Soc Med. 1991;84:179. doi: 10.1177/014107689108400327. [ DOI ] [ PMC free article ] [ PubMed ] [ Google Scholar ]
- 22. Condon RE. Curious intersections of bugs and bees. Surg. 1993;113:234–235. [ PubMed ] [ Google Scholar ]
- 23. Osato MS, Reddy SG, Graham DY. Osmotic effect of honey on growth and viability of Helicobacter pylori. Dig Dis Sci. 1999;44:462–464. doi: 10.1023/a:1026676517213. [ DOI ] [ PubMed ] [ Google Scholar ]
- 24. White JW, et al. Composition of American honeys. USDA Tech Bull. 1962. p. 1261.
- 25. Ali AT, Chowdhury MN, Al Humayyd MS. Inhibitory effect of honey on Helicobacter pylori Trop Gastroenterol. 1991;12:139–143. [ PubMed ] [ Google Scholar ]
- 26. Scrimgeour EM, Alexander PC, Rafay Akbar, Al-Riyami Kassin. Antibiotic Handbook. 6th Ed. Oman: Courtesy of Glaxo Smith Kline; 2003. [ Google Scholar ]
- 27. McLoughlin R, Racz I, Buckley M, O’Connor HJ, O’Morain C. Therapy of Helicobacter pylori. Helico. 2004;9:42–49. doi: 10.1111/j.1083-4389.2004.00251.x. [ DOI ] [ PubMed ] [ Google Scholar ]
- 28. Da Mota Menezea V, Atallah AN, Lapa AJ, Catapani WR. Assessing the therapeutic use of Lafoensia pacari St. Hil. Extract (Mangavabrava) in the eradication of Helicobacter pylori: double blind randomized clinical trial Helico. 2006;11:188–95. doi: 10.1111/j.1523-5378.2006.00399.x. [ DOI ] [ PubMed ] [ Google Scholar ]
- 29. Pantoflickova D, Corthesy-Theulaz I, Dorta G, et al. Favourable effect of regular intake of fermented milk containing Lactobacillus johnsonii on Helicobacter pylori associated gastritis. Aliment Pharmacol Ther. 2003;18:805–813. doi: 10.1046/j.1365-2036.2003.01675.x. [ DOI ] [ PubMed ] [ Google Scholar ]
- 30. Di Mario F, Aragona G, Bo ND, Ingegnoli A, Cavestro GM, et al. Use of lactoferin for Helicobacter pylori eradication. Aliment Pharmacol Ther. 2003;17:125–129. [ Google Scholar ]
- 31. Boyanova L, Dereji S, Koumanova R, Katsarov N, Gergova G, Mitov I, Nikolov R, Krastev Z. Inhibition of Helicobacter pylori growth in vitro by Bulgarian propolis: preliminary report. J Med Microbiol. 2003;52:417–419. doi: 10.1099/jmm.0.04895-0. [ DOI ] [ PubMed ] [ Google Scholar ]
- 32. Mahady GB, Pendland SL, Yun GS, Lu ZZ, Stoia A. Ginger (Zingiber officinale Roscoe) and the gingerols inhibit the growth of cagA+ strains of Helicobacter pylori. Anticancer Res. 2003;23:3699–3702. [ PMC free article ] [ PubMed ] [ Google Scholar ]
- 33. Galan MV, Kishan AA. Silverman Al Oral broccoli sprouts for the treatment of Helicobacter pylori infection: A preliminary Report. Dig Dis Sci. 2004;49:1088–1090. doi: 10.1023/b:ddas.0000037792.04787.8a. [ DOI ] [ PubMed ] [ Google Scholar ]
- 34. Al Jabri AA, Al Hosni SA, Nzeako BC, Al Mahrooqi ZH, Nsanze H. Antibacterial activity of Omani honey alone and in combination with gentamicin. Saudi Med J. 2005;26:767–771. [ PubMed ] [ Google Scholar ]
- PDF (815.6 KB)
- Collections
Similar articles
Cited by other articles, links to ncbi databases.
- Download .nbib .nbib
- Format: AMA APA MLA NLM
Add to Collections
An official website of the United States government
Official websites use .gov A .gov website belongs to an official government organization in the United States.
Secure .gov websites use HTTPS A lock ( Lock Locked padlock icon ) or https:// means you've safely connected to the .gov website. Share sensitive information only on official, secure websites.
- Publications
- Account settings
- Advanced Search
- Journal List

The Antibacterial Effect In Vitro of Honey Derived from Various Danish Flora
Reem dina matzen, julie zinck leth-espensen, therese jansson, dennis sandris nielsen, marianne n lund, steen matzen.
- Author information
- Article notes
- Copyright and License information
Academic Editor: Bruno A. Bernard
Corresponding author.
Received 2018 Mar 16; Revised 2018 May 21; Accepted 2018 May 24; Collection date 2018.
This is an open access article distributed under the Creative Commons Attribution License, which permits unrestricted use, distribution, and reproduction in any medium, provided the original work is properly cited.
The mechanism behind the biologic actions of honey as a wound remedy has been intensively studied; however, there is no published data regarding any antibacterial effect of honey derived from Danish flora. We surveyed 11 honeys of various Danish floral sources for their antibacterial activity and compared them to a culinary processed commercial honey (Jakobsens) and a raw and a medical grade Manuka ( Leptospermum scoparium ) honey using the agar-well diffusion method. We tested the effect on three gram-positive bacteria (two strains of Staphylococcus aureus and one strain of Staphylococcus epidermidis ) and two gram-negative bacteria ( Pseudomonas aeruginosa and Escherichia coli ). All samples, except the commercial honey, exhibited antibacterial activity, and samples derived from Water Mint ( Mentha aquatica), Organic 2 (mixed organic flora), and Linden ( Tilia cordata ) honey had consistent effects on all bacteria tested and showed greater effect than medical grade and raw Manuka ( L. scoparium ) honey. The content of methylglyoxal was low in the Danish honey (< 2 μ g/mL) and significantly (p<0.05) higher in both the raw and the medical grade Manuka ( L. scoparium ) honey, where the concentrations were, respectively, 6.29 μ g/mL and 54.33 μ g/mL. The antibacterial effect of Danish honeys was mostly due to hydrogen peroxide. We conclude that honeys derived from Danish flora possess antibacterial effect, probably by a hurdle effect of viscosity, osmolality, acidity, bioactive peptides, and most importantly the content of hydrogen peroxide. These findings indicate that honeys of various Danish floral sources may have clinical potential, although further studies are necessary to elucidate this in order to determine whether the results of our in vitro experiments also apply to a clinical setting.
1. Introduction
Honey has drawn increasing attention as a remedy for wound treatment of different kinds, mainly due to a verified antibacterial activity [ 1 ]. Antibiotic resistance and chronic wound infections have increased the interest in antimicrobial treatments, including honey-based wound care products, and these have been registered with medical regulatory authorities as wound care agents in many countries, among others, the European Union, USA, and New Zealand. These products are mainly based on Manuka ( Leptospermum scoparium ) honey from New Zealand.
Honey is a collection of nectar and consists of sugar (75–79%), water (20%), proteins, vitamins, minerals, and antioxidants [ 2 ]. The mechanisms of action of honey have been studied intensively, and it is acknowledged that it exerts a wound healing effect through a series of physical and bioactive properties [ 1 ]. The antibacterial activity of honey can be attributed to the natural occurrence of the enzymatic production of hydrogen peroxide (H 2 O 2 ) and a varied presence of phytochemical components such as methylglyoxal (MGO) [ 3 , 4 ]. The concentration of H 2 O 2 in honey is low while still having a disinfectant and tissue debridement effect without being cytotoxic and causing tissue damage [ 5 ]. Furthermore, honey has a low pH, high osmolality, and viscous properties, which inhibits the growth of microorganisms [ 6 ].
Honey of different geographical and floral origins may possess differences in antibacterial properties, which may be related to different chemical compositions of honeys [ 5 , 6 ]. Honey derived from the Leptospermum species in New Zealand (Manuka) and Australia is characterized by a high antibacterial activity even in the presence of catalase, which is an enzyme destroying H 2 O 2 . This nonperoxide activity is attributed to the high concentration of MGO, which is derived from dihydroxyacetone, present in large amount in the nectar from Leptospermum species [ 5 , 7 ]. But other factors, such as phytochemical substances and polypeptides like bee-defensin-1, may contribute to the overall biological effects of honey as well [ 8 , 9 ].
The raw Danish honeys which are sold via farmer's markets or local shops are characterized by not being heated in the process of production. This is mainly due to the fact that the raw Danish honey submerges from local beekeepers that process the honey immediately in glasses ready for sale, unlike commercial honeys, which are heated in order to liquefy after being stored. This immediate processing without heating preserves the natural enzymatic properties of the honey, but there is also a small risk of bacterial contamination. In order to not jeopardize the antibacterial effect of honey and to eradicate microorganisms, such as Clostridium botulinum spores which are sometimes found in honey, medical grade honey should be sterilized by gamma-irradiation, and not by heating which destroys the enzyme glucose oxidase [ 10 , 11 ].
Interestingly, there is no published data regarding any antibacterial effect of honey derived from Danish flora, despite a large tradition for production and sale of raw honey in Denmark and the obvious interest in developing a local, biological, and therapeutically useful remedy for wound care.
The aim of this study was to examine the biological activities of Danish honey of different floral sources, determine the antibacterial activity of the various types in vitro , and correlate this with the presence of H 2 O 2 and MGO. Furthermore, we analyzed a commercial (heated) honey and a medical grade Manuka ( L. scoparium ) honey as well as a raw Manuka ( L. scoparium ) honey from New Zealand for comparison.
2. Material and Methods
2.1. honey samples.
Honey samples of different floral sources ( Table 1 ) were collected from local beekeepers between July and August 2016. In addition, one sample was a commercial culinary processed honey from a Danish manufacturer Jakobsens and consisted of a blend of acacia honeys originating from different areas in Eastern Europe. Also, a raw Manuka ( L. scoparium ) honey was obtained from a local producer from New Zealand and a commercial medical grade Manuka ( L. scoparium ) honey ( “Activon”, Advancis Medical ) was included. All samples were stored in sterilized containers in the dark at room temperature (20-22°C). All tests were performed blinded, and labels were given after the experimental work and statistical analysis were completed. The source of floral identity was provided by the beekeepers based on availability of different sources for the nectar at the time of collection, the location of the apiary, and the organoleptic properties of the honey. The samples were diluted for handling by adding sterile Milli-Q water at 37°C to reach the desired dilutions. Throughout the antibacterial experiments, a solution of 75% honey was used, unless otherwise stated.
Honey samples included in the study. The Danish samples were obtained by two local beekeepers from the Zealand Region. The Activon Manuka is a medical grade honey and was obtained from Advancis Medical. The raw Manuka was obtained from a local beekeeper in New Zealand.
2.2. Pathogens
The pathogens (Culture collection of Food Microbiology and Fermentation, Department of Food Science at University of Copenhagen, Denmark) used for this study included
Staphylococcus aureus CCUG 1800;
Staphylococcus aureus 1094-7;
Staphylococcus epidermidis CCUG 39508;
Pseudomonas aeruginosa SKN 1317;
Escherichia coli K 12.
2.3. Procedure and Measurement
For testing the antibacterial effects of the honey samples the agar-well diffusion method was applied using Brain Heart Infusion (BHI) broth (CM0113) and BHI Agar (CM1136) as medium, prepared according to the instructions of the manufacturer (OXOID Ltd. Basingstoke, Hampshire, England).
The BHI agar was heated in microwave, then cooled to 50°C, and transferred into sterile tubes of 30 mL agar and 100 μ L of the tested pathogen (propagated in BHI broth overnight). The agar was stirred before pouring into sterile Petri-dishes and kept to solidify at room temperature for 30 min.
Wells (5 mm) were cut into the agar-dishes and 50 μ L of the honey samples was pipetted into each well. Milli-Q water served as reference. The dishes were incubated at 37°C for 48 h and the diameters of the growth inhibition zones were measured in centimeters to the nearest 0.05 cm.
To identify the primary antibacterial substance of the honey, following tests were performed: osmotic stress, dilution of the samples, thermal sensitivity, the effect of MGO, and enzyme sensitivity. All experiments were performed with duplicate samples of the honey, unless otherwise stated.
2.4. Osmotic Effect
A sample of 75% sucrose (w/w), corresponding to the amount of sugar in honey, was made by diluting 7.5 g sucrose in 2.5 g sterile Milli-Q water. A sample of 15% sucrose (w/w), corresponding to the amount of sugar in 20% honey samples, was made by diluting 1.5 g sucrose into 8.5 g sterile Milli-Q water. 50 μ l of the pure sugar samples was placed in the agar wells and procedure followed as described above.
2.5. Testing Thermal Sensitivity
500 μ L of each sample was added to Eppendorf tubes and heated in either a 60°C water bath or in a pot with boiling water (100°C) for 30 min. before testing.
2.6. Testing the Effect of Methylglyoxal
Two dilutions of 40% methylglyoxal in water solution (CAS 78-98-8, SIGMA-ALDRICH) were prepared. For a 0.02% concentration (200 μ g/mL), 10 μ l methylglyoxal was added to 19.99 mL sterilized water. For a 0.04% concentration (400 μ g/mL), 20 μ l methylglyoxal was added to 19.98 mL sterilized water.
2.7. Testing Enzyme Sensitivity
The honey samples were treated with two different enzymes, proteinase-K and catalase, to investigate the significance of a possible bioactive polypeptide and H 2 O 2 . 500 μ l of each honey sample (75% honey) was pipetted into two Eppendorf tubes. One sample was added 50 μ l of a 10 mg/ml proteinase-K solution K (CAS No. 39450-01-6, SIGMA-ALDRICH) for a 1 mg/mL solution. The other 500 μ l of each honey sample was added 10 μ l of a 50 mg/ml catalase solution (CAS No. 9001-05-2, SIGMA-ALDRICH) for a 1 mg/mL solution.
All samples were incubated at 37°C for 2 hours before being filled in the wells and tested as described previously. The experiments were only carried out on four out of the five pathogens mentioned in Section 2.2 , thereby excluding Pseudomonas aeruginosa SKN 1317, due to no remarkably inhibiting activity in the previous experiments.
2.8. pH Measurement
pH measurements were carried out using a calibrated PHM250 Ion Analyzer-Radiometer Analytical.
2.9. Determination of MGO Concentration in Honeys
The determination of dicarbonyls in honey was performed according to Adams [ 12 ]. Briefly, 2 mL of Milli-Q water was mixed with 0.6 g of honey. Subsequently, 1.5 mL of the diluted honey was mixed with 0.75 mL 2% o -phenyl diamine (OPD) (98%; Sigma, Steinheim, Germany) in phosphate buffer (0.5 M, pH 6.5; Merck, Darmstadt, Germany), in triplicate, and left over night to derivatize (19 hours). After the derivatization, the samples were filtered through 0.2 μ m filters and analyzed by a method based on ultrahigh performance liquid chromatography (UHPLC) described by Hellwig et al. [ 13 ]. In short, the samples were separated on a Prontosil 60 phenyl material (250 mm ∗ 4.6 mm, 5 μ m), with a guard column (Knauer, Berlin Germany, 5 ∗ 4 mm) filled with the same material and an online filter (3 μ m). The injection volume was 50 μ L, flow rate was 0.7 mL/min, and the UV detection was 312 nm. Eluent A consisted of 0.075% acetic acid (Sigma, Steinheim, Germany), and eluent B was 80% methanol (Sigma, Steinheim, Germany) and 20% of eluent A. The gradient was as follows: 10% B to 50% B from 0 to 27 min. by a linear gradient, 50% B kept constant from 27 to 30 min., increase from 50% to 70% B from 30 to 34 min. by a linear gradient followed by an increase to 100% B to 44 min., which was kept constant from 44 to 48 min., and finally back to 10% B from 48 to 50 min. by a linear gradient. A standard curve was prepared with the quinoxaline of MGO (Sigma, Steinheim, Germany) in the range between 0.4 μ g/mL and 20 μ g/mL.
2.10. Statistical Analyses
As the distribution of data was assumed normal, the statistical analysis was carried out using Excel v15.26 and StatPlus 2016 v6.1.60 for t-tests and one-way ANOVA tests. In addition, measurements of least squares means (lsmeans) were applied on the data for pairwise comparison of the MGO data (alfa=0.05) by the software RStudio (RStudio Team (2015), version 0.99.446, RStudio: Integrated development for R. RStudio, Inc., Boston, MA).
All the Danish honeys had antibacterial effect (p<0.05), and the honey samples Organic 2 (mixed organic flora), Water Mint ( Mentha aquatica ), and Linden ( Tilia cordata ) even possessed specific activity against E. coli and P. aeruginosa , while the medical grade Manuka ( L. Scoparium ) honey showed no activity at all towards these species. There were no significant differences (p>0.05) between the duplicate testing of all samples and all controls did not show any significant value throughout the various experiments. An example of the test results after agar diffusion method is presented in Figure 1 .
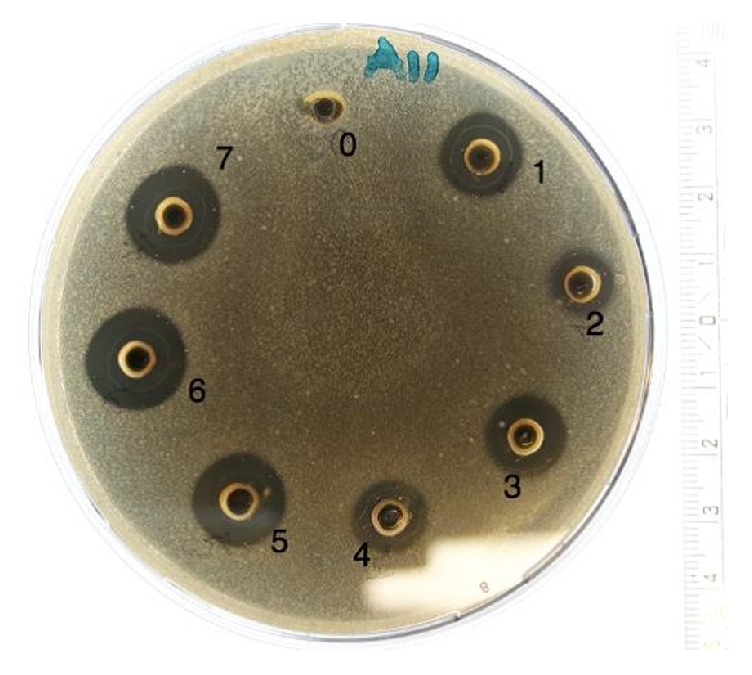
Photo of an agar-dish after agar-well diffusion assay. The dish is inoculated with Staphylococcus aureus (CCUG 1800) and honeys 1-7 are added in the wells. Milli-Q water serves as reference [0].
The bar charts in Figures 2 and 3 disclose the antibacterial effect of the honey samples. The Water Mint ( M. aquatica ), Linden ( T. cordata ), and Organic 2 (mixed organic flora) were able to inhibit all of the tested pathogens, showed the greatest inhibition zones and had a significant (p<0.05) effect on the gram-negative pathogens. The antibacterial effect of the honeys was greatest on the three gram-positive pathogens as compared to the gram-negative pathogens. The two honeys Hawthorn ( Crataegus monogyna ) and Activon Manuka ( L. scoparium ) showed the least inhibitory effect on the three gram-positive Staphylococci and were not able to inhibit the two gram-negative bacteria P. aeruginosa and E. coli . The commercial honey, Jakobsens, had no antibacterial effect in any of the tests.
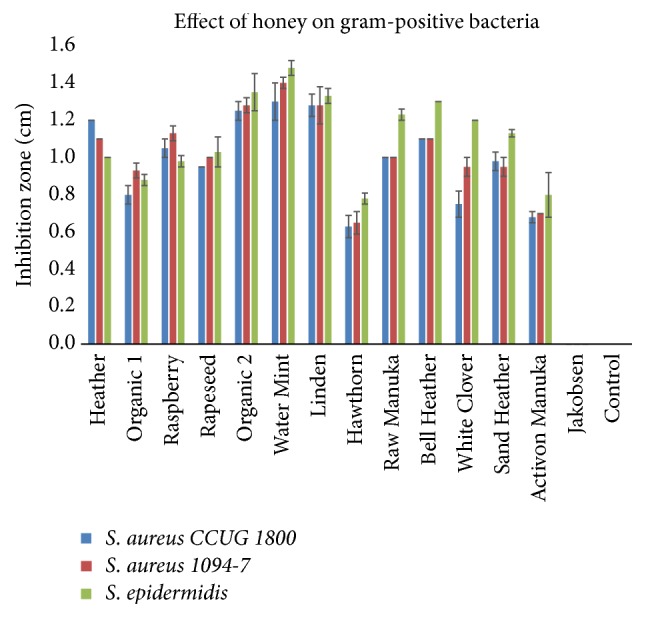
Effect of different floral sources of honey on growth of gram-positive bacteria (mean ± SD; n = 2).
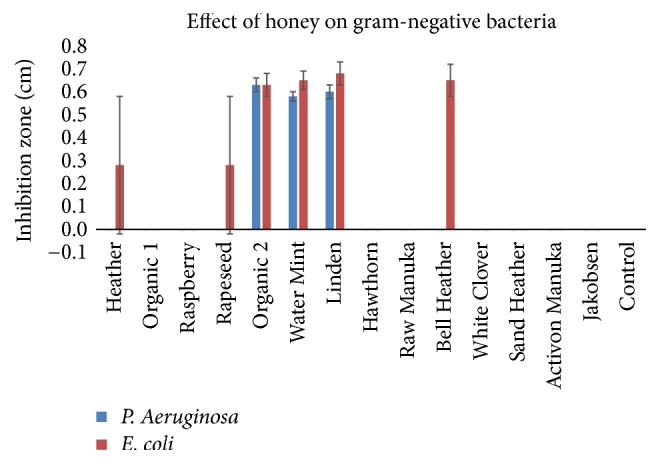
Effect of different floral sources of honey on growth of gram-negative bacteria (mean ± SD; n = 2).
3.1. Osmotic Effect
The pure sugar samples (75% and 15% sucrose) did not show any inhibition on the five selected pathogens.
3.2. Thermal Sensitivity
The heat treatment of the honey samples revealed a reduction in the inhibitory effect on the tested pathogens. The heat treatment of the honey samples at 100°C in 30 min. inhibited all antimicrobial effect in all honey samples; however, honey samples Heather ( Calluna vulgaris ), Raspberry ( Rubus odoratus ), Rapeseed ( Brassica napus ), Organic 2 (mixed organic flora), Water Mint ( M. aquatica), and Linden ( T. cordata ) were able to inhibit microbial growth of some of the pathogens after heat treatment at 60°C ( Figure 4 ).
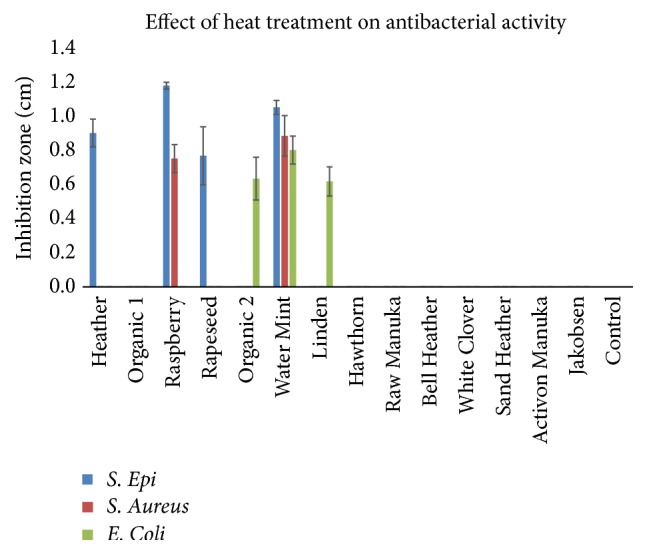
Antimicrobial effect of honey samples after heat treatment at 60°C/30 min. Some samples still showed antibacterial activity. These samples include Heather, Raspberry, Rapeseed, Organic 2, Water Mint, and Linden (mean ± SD; n = 2).
3.3. Effect of MGO
Pure MGO solutions containing 200 μ g/mL or 400 μ g/mL showed an inhibitory effect on four out of the five tested pathogens, as P. aeruginosa samples were excluded from the study due to contamination. At a concentration of 400 μ g/mL MGO, an inhibitory effect was seen on all four tested pathogens and significantly (p<0.05) higher compared to the 200 μ g/mL MGO in three of the tested pathogens ( S. aureus (1094-7), S. epidermidis , and E. coli ).
3.4. Effect of Proteinase-K
All samples showed varying inhibitory effects on the different bacteria. For S. aureus (1094-7), honey samples Organic 1 (mixed organic flora), Rapeseed ( B. napus ), Water Mint ( M. aquatica ), Hawthorn ( C. monogyna ), and Bell Heather ( Erica tetralix ) had significantly (p<0.05) reduced activity after proteinase-K treatment. For S. epidermidis, comparable results were observed with Raspberry ( R. odoratus ), Hawthorn ( C. monogyna ), and Bell Heater ( E. tetralix ) (p<0.05). For E. coli , proteinase-K treatment resulted in a significant (p<0.05) decrease in nine of the 13 honey samples, and in six of the samples the inhibitory effect was lost completely ( Table 2 ).
Mean zones of inhibition (mm) before (-) and after (+) treatment with the proteolytic enzyme proteinase-K on the 14 different honey samples.
The antibacterial effect of different honey types on four different bacteria was assessed by the agar well diffusion method on duplicate samples and the mean ± SD are presented.
Symbol (-) indicates insufficient samples (n=1 or 0).
Symbol ( ∗ ) denotes statistically significance (p< 0.05; enzyme treatment versus no treatment).

3.5. Effect of Catalase
Treatment with catalase abolished the antimicrobial effect of all the Danish honey samples, while the Activon Manuka ( L. scoparium ) maintained a significant antibacterial effect on S. aureus (1094-7) and epidermidis (p<0.05) ( Table 3 ).
Mean zones of inhibition (mm) before (-) and after (+) treatment with the enzyme catalase on the 14 different honey samples.
3.6. pH in Honey
The 14 honey samples had a pH varying between 3.25 and 3.77 (mean: 3.49 and standard variation: 0.14) ( Figure 5 ).
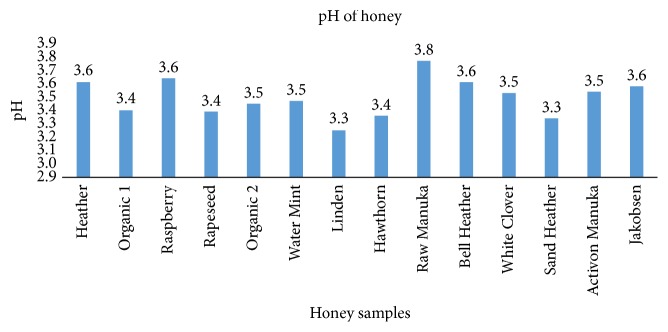
pH values of the 14 tested honey samples. The measurements are performed on a solution of 20% honey ( n = 1).
3.7. Methylglyoxal in Honey Samples
The concentrations of MGO in Activon Manuka ( L. scoparium ) and in raw Manuka honey ( L. scoparium ) were, respectively, 54.33 μ g/mL and 6.29 μ g/mL, and these concentrations were significantly higher than the other honey samples ( Figure 6 ).
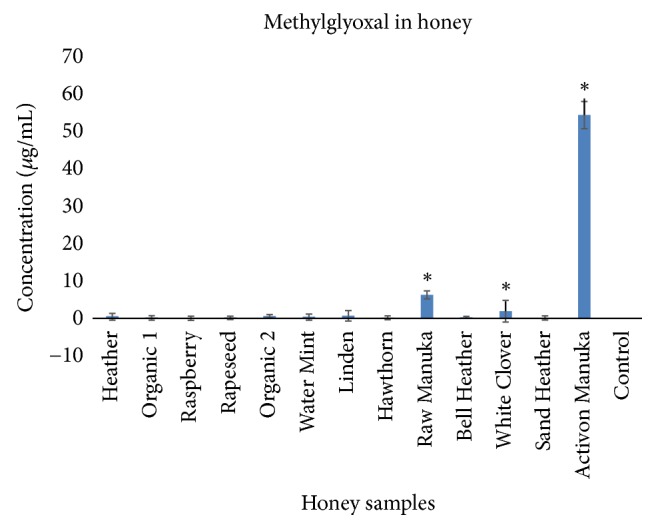
Concentration of methylglyoxal in 13 of the tested honey samples (mean ± SD, n=3). Symbol ( ∗ ) denotes statistical difference (p<0.05).
4. Discussion
This is the first study demonstrating that honey derived from Danish flora exhibit antimicrobial effects. This biological effect was for some honeys similar to or higher than the antibacterial effect of Manuka ( L. scoparium ) honey, especially regarding the inhibition of gram-negative microorganisms. The mechanism of action is mainly due to the content of H 2 O 2 present in the Danish honey. The content of MGO is low in the Danish honeys compared to the medical grade Manuka ( L. scoparium ) honey, and the influence of this phytochemical substance on the antimicrobial effect of Danish honeys is probably of minor significance.
Clear differences could be observed in the antibacterial effect of the floral sources of honey with the Water Mint ( M. aquatica ), Linden ( T. cordata ), and Organic 2 (mixed organic flora) having the most consistent antibacterial effects on all tested pathogens. Also, the commercial culinary processed honey does not show any inhibition of the pathogens. This result, in conjunction to the results from the pure sugar samples, indicates that the antimicrobial effect is not simply conditional to the sugar content in the honey.
The gram-positive target strains were the most susceptible to honey whereas the gram-negative microbes were less sensitive to all honey samples including Manuka ( L. scoparium ), which is in accordance with previous observations [ 9 , 14 ]. The difference in susceptibility to honey and other antibacterial agents between gram-positive and gram-negative microbes may be due to the composition of the cell wall. Gram-positive bacteria do not have an outer membrane protecting the peptidoglycan layer in contrast to gram-negative bacteria making it easier for antimicrobial agents to penetrate and cause damage [ 15 ].
The production of H 2 O 2 by the presence of the enzyme glucose oxidase in honey is considered to be an important factor for the overall antibacterial effect of honeys [ 16 ]. But the concentration does not reach levels that are considered cytotoxic [ 17 ], which could be of relevance in case of applying honey as a remedy for wound care [ 18 ]. In the present study it was observed that treating the honey samples with catalase, an enzyme inhibiting glucose oxidase and thereby the production of H 2 O 2 , significantly reduced the antibacterial effect of all the Danish honeys. However, the antibacterial effect of the Manuka ( L. scoparium ) honey on some of the gram-positive strains was unaffected. This finding is in accordance with previous observations that more important factors than the level of H 2 O 2 accounts for the antibacterial effect of this sort of honey [ 1 , 19 ]. It was confirmed that MGO, at least in the higher concentration (400 μ g/mL), had an antibacterial effect, and together with the finding of a high level of MGO in the Manuka ( L. scoparium ) honey samples, this substance can be accountable for most of the antibacterial effect of this honey as reported previously [ 19 ]. In contrast, the Danish honeys had very low MGO levels, which is why this substance has little or no significance to the antibacterial effects of these particular honey types. On the other hand, studies have indicated that honeys with H 2 O 2 -dependent activity may be more broad spectrum and therapeutically useful as antifungal agents than Manuka honey, because they were found to be more effective than Manuka honey at inhibiting dermatophyte fungi [ 20 ] and species of the yeast Candida [ 21 ].
Besides the production and content of H 2 O 2 or MGO, other properties of honey may contribute to the overall effects on bacteria. The low water content of honey and high osmolality and viscosity, acidic pH, and presence of leptosin and polypeptides like bee-defensin-1 have all been found to contribute to the reduced bacterial growth [ 22 ]. The low pH of honey did not seem to be an important factor in the present study. High osmolality is presumed to add to the antimicrobial effect of honey. However, from our experiments, high concentrations of glucose alone did not show any inhibition of bacterial growth. Furthermore, the heated preprocessed commercial honey had no antibacterial effect at all, despite a presumed high level of sugar. Other studies also indicate that solutions of glucose has less antimicrobial effect than honey [ 23 ], and the sugar-induced osmolality in honey is merely regarded as contributing to an unfavorable environment for pathogens, rather than being a primary inhibiting factor on bacterial growth by itself.
For further characterization of the mechanisms behind the antibacterial effect observed in the Danish honeys, the involvement of bioactive peptides was investigated by adding proteinase-K to the different honey samples. Proteinase-K was expected to cleave the proteins and thereby inactivate bioactive peptides such as defensin-1 [ 5 ]. Defensins are antibacterial peptides created to protect the host cells from invasion and infection by pathogens [ 24 ]. We found overall smaller inhibition zones on the agar plates with some differences between pathogens tested and the honey types after application of proteinase-K. It has previously been reported that there are differences in the presence of bee-defensin-1 in different sorts of honeys [ 9 ]. Our study shows a reduced effect on bacterial growth after adding a proteolytic enzyme and this is indicative of involvement of a biologic active peptide. However, further studies and other methods are necessary to elucidate if Danish honeys contain bioactive bee-defensin-1.
Throughout the experiments, the agar-well diffusion method was applied and used with a 75% solution of honey, for practical reasons, to determine the antibacterial effects of the honey samples. The 75% solution of honey had significant effect on the bacterial growth, while no effect was seen for the 20% solution of honey. This finding was also applied for the Manuka ( L. scoparium ) honey. It is well established that the agar-well diffusion method is suited for testing antibacterial effect, but if minimum inhibitory concentrations (MIC) are to be calculated, a more sensitive method like agar or broth dilution, where the honey is incorporated directly into the agar growth media must be used [ 25 ]. By this method the microbes are brought into direct contact with the testing inhibiting substance, and thereby not relying on the agent's ability to diffuse through the agar media [ 26 ].
Due to the physical characteristics of honey, it may contribute to a moist environment, which is beneficial for wound healing [ 27 ]. Furthermore, honey has been shown to stimulate the immune response and reduce inflammation, which in turn leads to an accelerated wound healing [ 1 , 28 , 29 ]. In addition, honey may also reduce the need for surgical wound debridement in selected cases [ 18 , 30 ]. However, if honey is to be applied as a medical remedy for wound care it is necessary to process the honey for sterilization in order to eliminate a possible presence of pathogens or C. botulinum spores [ 11 ], why sterilization by gamma-irradiation should be performed [ 10 ]. No significant change in the antibacterial activity of honey was found caused by this method of sterilization of honey, neither in the honeys with H 2 O 2 -dependent activity or in the Manuka honeys [ 10 ]. Additionally, no significant changes were found in the physiochemical and mineral contents of honey resulting from sterilization by gamma-irradiation [ 31 ]. The process of heating honey will eliminate pathogens but also reduce the activity of H 2 O 2 and other antibacterial substances. This is verified in the present study where the antibacterial activity was inhibited in most of the samples by heating the honey to 60°C.
5. Conclusion
This is the first study providing a substantial in vitro investigation of the antibacterial effect of honey derived from various Danish flora. We verified great variation in different floral sources with the Water Mint ( M. aquatica ), Linden ( T. cordata ), and Organic 2 (mixed organic flora) possessing the highest antibacterial activity on all the tested pathogens. These Danish honeys were comparable and even superior to commercial medical grade honey. The antibacterial effect was probably due to the activity of H 2 O 2 , though no direct measurements of the concentration of this substance was performed. Other studies have also been able to verify variation in antibacterial activity of honey depending on geographical location and floral source [ 26 , 32 ].
Since the foraging of bees is not completely controllable and depends on the dominant floral source at the time of collection, it will be almost impossible to standardize a natural monofloral honey. Therefore, it is reasonable to assume that a specific floral source of honey also builds on a certain percentage of nonspecific nectar. However, while the antibacterial activity of honey might be a result of a hurdle effect of the honey's phytochemical characteristics, pH, viscosity, and content of H 2 O 2 , the mixture of different honey types might prove superior to a monofloral honey. Further studies are necessary to elucidate this hypothesis and to determine whether the results of our in vitro experiments also apply to a clinical setting.
Data Availability
The data used to support the findings of this study are available from the corresponding author upon request.
This research did not receive any specific grant from funding agencies in the public, commercial, or not-for-profit sectors.
Conflicts of Interest
The authors report no conflicts of interest.
Authors' Contributions
All authors have contributed equally to the above.
- 1. Molan P., Rhodes T. Honey: a biologic wound dressing. Wounds. 2015;27(6):141–151. [ PubMed ] [ Google Scholar ]
- 2. Bansal V., Medhi B., Pandhi P. Honey—a remedy rediscovered and its therapeutic utility. Kathmandu University Medical Journal. 2005;3(3):305–309. [ PubMed ] [ Google Scholar ]
- 3. Alam F., Islam M. A., Gan S. H., Khalil M. I. Honey: a potential therapeutic agent for managing diabetic wounds. Evidence-Based Complementary and Alternative Medicine. 2014;2014:16. doi: 10.1155/2014/169130.169130 [ DOI ] [ PMC free article ] [ PubMed ] [ Google Scholar ]
- 4. Jenkins R., Burton N., Cooper R. Proteomic and genomic analysis of methicillin-resistant staphylococcus aureus (MRSA) exposed to manuka honey in vitro demonstrated down-regulation of virulence markers. Journal of Antimicrobial Chemotherapy. 2014;69(3):603–615. doi: 10.1093/jac/dkt430. [ DOI ] [ PMC free article ] [ PubMed ] [ Google Scholar ]
- 5. Irish J., Blair S., Carter D. A. The antibacterial activity of honey derived from Australian flora. PLoS ONE. 2011;6(3) doi: 10.1371/journal.pone.0018229.e18229 [ DOI ] [ PMC free article ] [ PubMed ] [ Google Scholar ]
- 6. Moussa A., Noureddine D., Mohamed H. S., Abdelmelek M., Saad A. Antibacterial activity of various honey types of Algeria against Staphylococcus aureus and Streptococcus pyogenes. Asian Pacific Journal of Tropical Medicine. 2012;5(10):773–776. doi: 10.1016/S1995-7645(12)60141-2. [ DOI ] [ PubMed ] [ Google Scholar ]
- 7. Allen K. L., Molan P. C., Reid G. M. A survey of the antibacterial activity of some New Zealand honeys. Journal of Pharmacy and Pharmacology. 1991;43(12):817–822. doi: 10.1111/j.2042-7158.1991.tb03186.x. [ DOI ] [ PubMed ] [ Google Scholar ]
- 8. Kwakman P. H. S., Te Velde A. A., De Boer L., Speijer D., Vandenbroucke-Grauls C. M. J. E., Zaat S. A. J. How honey kills bacteria. The FASEB Journal. 2010;24(7):2576–2582. doi: 10.1096/fj.09-150789. [ DOI ] [ PubMed ] [ Google Scholar ]
- 9. Kwakman P. H. S., De Boer L., Ruyter-Spira C. P., et al. Medical-grade honey enriched with antimicrobial peptides has enhanced activity against antibiotic-resistant pathogens. European Journal of Clinical Microbiology & Infectious Diseases. 2011;30(2):251–257. doi: 10.1007/s10096-010-1077-x. [ DOI ] [ PMC free article ] [ PubMed ] [ Google Scholar ]
- 10. Molan P. C., Allen K. L. The effect of gamma-irradiation on the antibacterial activity of honey. Journal of Pharmacy and Pharmacology. 1996;48(11):1206–1209. doi: 10.1111/j.2042-7158.1996.tb03922.x. [ DOI ] [ PubMed ] [ Google Scholar ]
- 11. Lusby P. E., Coombes A., Wilkinson J. M. Honey: a potent agent for wound healing? Journal of Wound Ostomy & Continence Nursing. 2002;29(6):295–300. doi: 10.1067/mjw.2002.129073. [ DOI ] [ PubMed ] [ Google Scholar ]
- 12. Adams M. R., Moss M. O. Food Microbiology. 3rd. RCS Publishing; 2008. [ Google Scholar ]
- 13. Hellwig M., Degen J., Henle T. 3-Deoxygalactosone, a 'new' 1,2-dicarbonyl compound in milk products. Journal of Agricultural and Food Chemistry. 2010;58(19):10752–10760. doi: 10.1021/jf102388v. [ DOI ] [ PubMed ] [ Google Scholar ]
- 14. Mandal M. D., Mandal S. Honey: its medicinal property and antibacterial activity. Asian Pacific Journal of Tropical Biomedicine. 2011;1(2):154–160. doi: 10.1016/s2221-1691(11)60016-6. [ DOI ] [ PMC free article ] [ PubMed ] [ Google Scholar ]
- 15. Madigan M., Martinko J., Bender K., Buckley D., Stahl D. Brock Biology of Microorganism. 14th. Pearson; 2015. [ Google Scholar ]
- 16. Brudzynski K. Effect of hydrogen peroxide on antibacterial activities of Canadian honeys. Canadian Journal of Microbiology. 2006;52(12):1228–1237. doi: 10.1139/W06-086. [ DOI ] [ PubMed ] [ Google Scholar ]
- 17. Bang L. M., Buntting C., Molan P. The effect of dilution on the rate of hydrogen peroxide production in honey and its implications for wound healing. The Journal of Alternative and Complementary Medicine. 2003;9(2):267–273. doi: 10.1089/10755530360623383. [ DOI ] [ PubMed ] [ Google Scholar ]
- 18. Dina Jarjis R., Thomas Crewe B., Henrik Matzen S. Post-bariatric abdominoplasty resulting in wound infection and dehiscence—conservative treatment with medical grade honey: a case report and review of literature. International Journal of Surgery Case Reports. 2016;20:1–3. doi: 10.1016/j.ijscr.2015.12.051. [ DOI ] [ PMC free article ] [ PubMed ] [ Google Scholar ]
- 19. Atrott J., Henle T. Methylglyoxal in manuka honey—correlation with antibacterial properties. Czech Journal of Food Sciences. 2009;27:S163–S165. doi: 10.17221/911-CJFS. [ DOI ] [ Google Scholar ]
- 20. Brady N. F., Molan P. C., Harfoot C. G. The sensitivity of dermatophytes to the antimicrobial activity of manuka honey and other honey. Journal of Pharmaceutical Sciences. 1996;2(10):471–473. [ Google Scholar ]
- 21. Irish J., Carter D. A., Shokohi T., Blair S. E. Honey has an antifungal effect against Candida species. Medical Mycology. 2006;44(3):289–291. doi: 10.1080/13693780500417037. [ DOI ] [ PubMed ] [ Google Scholar ]
- 22. Maddocks S. E., Jenkins R. E. Honey: a sweet solution to the growing problem of antimicrobial resistance? Future Microbiology. 2013;8(11):1419–1429. doi: 10.2217/fmb.13.105. [ DOI ] [ PubMed ] [ Google Scholar ]
- 23. Al-Waili N. S. Investigating the antimicrobial activity of natural honey and its effects on the pathogenic bacterial infections of surgical wounds and conjunctiva. Journal of Medicinal Food. 2004;7(2):210–222. doi: 10.1089/1096620041224139. [ DOI ] [ PubMed ] [ Google Scholar ]
- 24. Roberts A., Brown H. L., Jenkins R. On the antibacterial effects of manuka honey: mechanistic insights. Research and Reports in Biology. 2015;2015(6):215–224. doi: 10.2147/rrb.s75754. https://www.dovepress.com/on-the-antibacterial-effects-of-manuka-honey-mechanistic-insights-peer-reviewed-article-RRB , 2017. [ DOI ] [ Google Scholar ]
- 25. Balouiri M., Sadiki M., Ibnsouda S. K. Methods for in vitro evaluating antimicrobial activity: a review. Journal of Pharmaceutical Analysis. 2016;6(2):71–79. doi: 10.1016/j.jpha.2015.11.005. [ DOI ] [ PMC free article ] [ PubMed ] [ Google Scholar ]
- 26. Lusby P. E., Coombes A. L., Wilkinson J. M. Bactericidal activity of different honeys against pathogenic bacteria. Archives of Medical Research. 2005;36(5):464–467. doi: 10.1016/j.arcmed.2005.03.038. [ DOI ] [ PubMed ] [ Google Scholar ]
- 27. Junker J. P., Kamel R. A., Caterson E. J., Eriksson E. Clinical impact upon wound healing and inflammation in moist, wet, and dry environments. Advances in Wound Care. 2013;2(7):348–356. doi: 10.1089/wound.2012.0412. [ DOI ] [ PMC free article ] [ PubMed ] [ Google Scholar ]
- 28. Subrahmanyam M. A prospective randomised clinical and histological study of superficial burn wound healing with honey and silver sulfadiazine. Burns. 1998;24(2):157–161. doi: 10.1016/S0305-4179(97)00113-7. [ DOI ] [ PubMed ] [ Google Scholar ]
- 29. Molan P. C. Re-introducing honey in the management of wounds and ulcers-theory and practice. Ostomy Wound Management. 2002;48(11):28–40. [ PubMed ] [ Google Scholar ]
- 30. Tahmaz L., Erdemir F., Kibar Y., Cosar A., Yalcýn O. Fournier's gangrene: report of thirty-three cases and a review of the literature. International Journal of Urology. 2006;13(7):960–967. doi: 10.1111/j.1442-2042.2006.01448.x. [ DOI ] [ PubMed ] [ Google Scholar ]
- 31. Hussein S. Z., Yusoff K. M., Makpol S., Yusof Y. A. M. Does gamma irradiation affect physicochemical properties of honey? La Clinica Terapeutica. 2014;165(2):e125–e133. doi: 10.7471/CT.2014.1695. [ DOI ] [ PubMed ] [ Google Scholar ]
- 32. Wilkinson J. M., Cavanagh H. M. A. Antibacterial activity of 13 honeys against Escherichia coli and Pseudomonas aeruginosa. Journal of Medicinal Food. 2005;8(1):100–103. doi: 10.1089/jmf.2005.8.100. [ DOI ] [ PubMed ] [ Google Scholar ]
Associated Data
This section collects any data citations, data availability statements, or supplementary materials included in this article.
Data Availability Statement
- View on publisher site
- PDF (1.1 MB)
- Collections
Similar articles
Cited by other articles, links to ncbi databases.
- Download .nbib .nbib
- Format: AMA APA MLA NLM
Add to Collections
ORIGINAL RESEARCH article
Honey’s ability to counter bacterial infections arises from both bactericidal compounds and qs inhibition.

- 1 Department of Surgery, Harvard Medical School and Massachusetts General Hospital, Boston, MA, USA
- 2 Shriners Burns Institute and Massachusetts General Hospital, Boston, MA, USA
- 3 IYAR, The Israeli Institute for Advanced Research, Rehovot, Israel
The ability of honey to kill bacterial pathogens in vitro and quickly clear even chronic or drug-resistant infections has been demonstrated by several studies. Most current research is focused on identifying the bactericidal compounds in honey, but the action of the compounds discovered is not sufficient to explain honey’s activity. By diluting honey to sub-inhibitory levels, we were able to study its impact on bacterial coordinated behavior, and discovered that honey inhibits bacterial quorum sensing (QS). Experiments to characterize and quantify honey’s effect on the QS networks of Pseudomonas aeruginosa revealed that low concentrations of honey inhibited the expression of MvfR, las , and rhl regulons, including the associated virulence factors. This research also establishes that inhibition of QS is associated with honey’s sugar content. Therefore, honey combats infections by two independent mechanisms acting in tandem: bactericidal components, which actively kill cells, and disruption of QS, which weakens bacterial coordination and virulence.
Introduction
A critical problem facing modern medicine is the emergence of many strains of antibiotic-resistant bacteria ( Tomasz, 1994 ; Arias and Murray, 2009 ; So et al., 2010 ). The most dangerous of these are multi-drug-resistant “superbugs,” strains impervious to almost all known antibiotics. One example is the human opportunistic pathogen Pseudomonas aeruginosa , a Gram-negative bacterium notorious for infecting immunocompromised individuals, the cause of many nosocomial acquired infections including those caused in burn and cystic fibrosis patients. In recent years, strains of P. aeruginosa have appeared that resist virtually all known drugs ( Aloush et al., 2005 ), a situation exacerbated by the fact that there are almost no new antibiotics against Gram-negative bacteria in the pipeline ( Cornelis, 2008 ; Freire-Moran et al., 2011 ).
Honey, known for its medicinal uses since ancient times ( Zumla and Luat, 1989 ), has attracted new attention in the fight against drug-resistant bacteria. It was found to be very effective against various clinical isolates of bacteria, and to boost the effect of current antibiotics when applied to antibiotic disks ( Abd-El Aal et al., 2007 ; Kwakman et al., 2008 ). Recent research has shown that when tested against clinical isolates of Staphylococcus aureus and P. aeruginosa , honey killed free-living cells in all strains tested ( Alandejani et al., 2009 ). More importantly it was capable of killing bacteria even in their highly resistant biofilm state, proving more effective than any single commonly used antibiotic (rifampin, cefazolin, oxacillin, vancomycin, azithromycin, fusidic acid, gentamicin, and linezolid were tested). In addition to attacking various pathogens in vitro , clinical studies have shown that honey enhances healing and eliminates even chronic or drug-resistant infections in vivo ( Efem, 1988 ). Honey was also reported to be more effective than standard treatments for patients with infected burns ( Wijesinghe et al., 2009 ).
Honey’s antimicrobial properties are still not fully understood. Bees produce honey from flower nectar by evaporating water and adding digestive enzymes ( Crane, 1975 ). The two largest constituents of honey are sugars (81%) and water (17%; White et al., 1962 ; Jeffrey and Echazarretta, 1996 ). The remaining 1–2% contains various enzymes and compounds, whose composition plays a significant role in honey’s bactericidal activity and varies widely depending on nectar source ( Molan, 1999 ). Attempts to identify the source of bactericidal activity has led to the discovery of molecules such as methylglyoxal and bee-defensin 1 ( Kwakman et al., 2010 ), but accurately characterizing their effects is difficult due to the large number of trace components and the possibility of combinatorial effects.
Quorum sensing (QS) is a term describing bacterial communication used by many bacterial species and its based on the production and detection of diffusible signal molecules ( Atkinson and Williams, 2009 ). These molecules trigger signaling cascades, resulting in collective changes in behavior. Inhibition of QS would disrupt defensive measures and regulation of virulence, both weakening an infection and making it much more vulnerable to bactericidal elements. Moreover, as QS is not essential to survival, a strategy to inhibit it would reduce virulence while minimizing selection for resistance.
Pseudomonas aeruginosa uses two known QS systems: (1) the acyl-homoserine-lactone (AHL) LasR/RhlR network ( Fuqua et al., 2001 ; Shiner et al., 2005 ) and (2) the 4-hydroxy-2-alkylquinolines (HAQs) MvfR regulatory network ( Gallagher et al., 2002 ; Déziel et al., 2004 ; Wade et al., 2005 ). MvfR is critical for full virulence and leads to the positive regulation of a wide variety of virulence factors, many of which are also influenced by RhlR and LasR. The bacterium uses these networks to modulate its virulence and respond to environmental cues ( Bassler, 1999 ; Déziel et al., 2005 ; Hazan et al., 2010 ).
Here we show that non-bactericidal concentrations of honey (6% or less) inhibit both known QS networks used by P. aeruginosa by inhibiting the expression of genes in the MvfR, Las, and Rhl networks and activation of associated virulence factors. Combined with tests of bactericidal effects, this suggests that honey’s ability to combat infections stems from two separate mechanisms: (1) bactericidal effect from unique molecules (which have not been conclusively identified) that are nectar source dependent, and (2) effects on QS systems that are associated with sugar content and independent of nectar source.
Materials and Methods
Two honeys of different bactericidal strength were used in these experiments. Local honey (LH) was harvested from a hive of Italian honeybees ( Apis mellifera ligustica ), as a representative of an average honey. Manuka honey (MH), made from the nectar of Leptospermum scoparium or L. polygalifolium , was purchased from Manuka Health New Zealand (grade MGO 550 or 550 mg/kg methylglyoxal, batch NO. 030308). MH is known for its unusually strong bactericidal properties ( Molan, 1999 ).
The honeys were either used raw or heat-treated for 20 min at 100°C to inactivate any enzymes present ( Ahern and Klibanov, 1985 ), almost entirely eliminating bactericidal activity.
In experiments requiring dilutions the concentrations typically used were 2, 4, or 6% in 5 mL of Luria–Bertani (LB) broth.
Pseudomonas aeruginosa strains used were wild-type PA14 ( Rahme et al., 1995 ) and derivative isogenic strains, including isogenic mutant pqsA ( Déziel et al., 2004 ) from the Rahme lab stock, Mass-General-Hospital, Boston, MA, USA. Two types of reporter genes were used: (1) a pqsA –GFP (ASV) fusion consisting of a pqsA promoter upstream to a short live GFP (ASV) to allow detection of changes in pqs operon expression ( Yang et al., 2007 ) and (2) fusions of the lacZ promoter to lasI and rhlI ( Cao et al., 2001 ; Déziel et al., 2004 , 2005 ). E. coli was the laboratory strain DH5α (NEB). All bacteria were grown in LB broth at 37°C.
LB broth was purchased from BD Diagnostics. M9 minimal media was prepared by adding 200 mL M9 salts (64 g Na 2 HPO 4 ·7H 2 O, 15 g KH 2 PO 4 , 2.5 g NaCl, 5 g NH 4 Cl in a total volume of 1000 mL distilled water), 2 mL of 1 M sterile MgSO 4 , 20 mL of 20% glucose, and 100 μL of 1 M sterile CaCl 2 to 700 mL of distilled water and adjusting the volume to 1000 mL.
Bacterial Zone of Inhibition
The zone of inhibition on bacterial lawns was measured to compare the bactericidal properties of different honeys. LB agar plates were inoculated with bacterial lawns by plating out 100 μL of overnight liquid culture. Single drops of honey (10 μL) were pipetted onto these lawns. The plates were incubated 24 h at 37°C and the resulting clear areas around the drops were compared. Gentamicin (60 μg/mL; Sigma) and double distilled water were used as positive and negative controls respectively.
Minimum Inhibitory Concentration and Minimum Bactericidal Concentration Determination and Bacterial Growth
The minimum inhibitory concentration (MIC) of honey was determined by growing bacteria in a 96-well plate. Each row of wells contained a different type of honey or sugar, with initial concentrations of 60% honey or sugar and serial twofold dilutions down the rows. After incubating overnight at 37°C with no shaking, the lowest concentration at which the broth remained clear was defined as the MIC. Media from the wells with no visible bacterial growth were plated onto LB plates to check for surviving bacterial cells, and the lowest concentration that did not result in bacterial colonies was defined as the minimum bactericidal concentration (MBC). These concentrations were then compared to determine relative efficacy of the honey and sugar solutions tested. The growth of bacteria in presence of different types of honey or sugar was assessed after 18 h by measuring the optical density (OD 600 nm) using a spectrophotometer (Genesys) at 600 nm. Bacterial growth was calculated and reported as percentage of treated/untreated cultures.
Pyocyanin Assay
Cultures were grown in 5 mL of media overnight in tubes on a roller at 37°C. The following day samples were taken and measured for OD 600 nm . For pyocyanin extraction, bacterial cells were removed from 1 mL of culture by centrifuging followed by addition of 1 mL chloroform to the supernatant. Pyocyanin was extracted by the addition of HCl (0.5%) and the upper reddish phase was collected and its OD was measured at 520 nm ( Hazan et al., 2010 ). Both OD’s were plotted as percentages of the control to account for differences in cell density.
pqsA –GFP Assay
A P. aeruginosa strain with a pqsA –GFP (ASV) fusion was grown in 96-well black microtiter plates as previously described ( Hazan et al., 2010 ). Honey and sugar solutions were added to the media in the wells to determine their impact on expression. The growth and fluorescence of the GFP (excitation at 485 nm and emission at 535 nm) were recorded using plate reader Infinite F200 (Tecan).
Liquid Chromatography/Mass Spectrometry of QS Signaling Molecules
The quantification of HAQ concentration in bacterial culture supernatants was performed by liquid chromatography/mass spectrometry (LC–MS) as described previously ( Lépine et al., 2003 ). The HAQs were separated on a C18 reverse-phase column connected to a mass spectrometer using a water/acetonitrile gradient. Positive electrospray in the MRM mode with 2 × 10 −3 mTorr argon and 30 V as the collision gas were employed to quantify HAQs using the ion transitions HHQ 244 > 159, HHQ-D4 248 > 163, HQNO 260 > 159, PQS 260 > 175, and PQS-D4 264 > 179. The pseudomolecular ions of each compound were monitored in full scan mode using the unsaturated PA14 HAQs response factors.
β-Galactosidase Activity Assay
PA14 or isogenic mutant cultures carrying pGX5 ( pqsA–lacZ ) were grown overnight at 37°C, diluted to OD 600 = 0.01, and grown at 37°C; and β-galactosidase activity was measured as described ( Miller, 1972 ) at different time points. Briefly, 0.9 mL of Z buffer was added to 0.1 mL of a liquid culture. A drop of SDS (0.1%) and two drops of Chloroform were added followed by 15′′ vortex and mixed with 0.2 mL of ONPG ( o -nitrophenyl-β- D -galactoside; 4 mg/mL). Tubes were then vortexed, incubated until sufficient yellow color had developed and measured for optical density at 420 and 550 nm. Results were expressed in Miller units (MU).
Protease Assay
Production of extracellular protease was tested using plates made with brain heart infusion (BHI) and skim milk ( Sokol et al., 1979 ). To test the effects of honey, 4% MH was mixed in before pouring. 2 μL of PA14 culture at OD 600 nm = 2.0. Plates were then incubated room temperature (about 24°C) for 48 h and resulting clear halos were measured.
Bactericidal Effect of Honey
Honeys have been shown to have strong bactericidal properties against many species of bacteria that commonly infect wounds ( Willix et al., 2008 ). In order to determine whether inhibition of QS is linked to this, we confirmed previous information on the nature of honey’s activity.
Firstly, it has been shown that the bactericidal effects of honey far exceed those of comparable sugar solutions ( Efem and Iwara, 1992 ). Our tests of MIC/MBC of honey relative to sugars revealed that the minimum concentration of glucose or fructose required to prevent growth of P. aeruginosa cells in liquid culture is roughly fourfold that of honey, as shown in Table 1 . This rules out osmotic stress as a deciding factor in antibacterial activity.
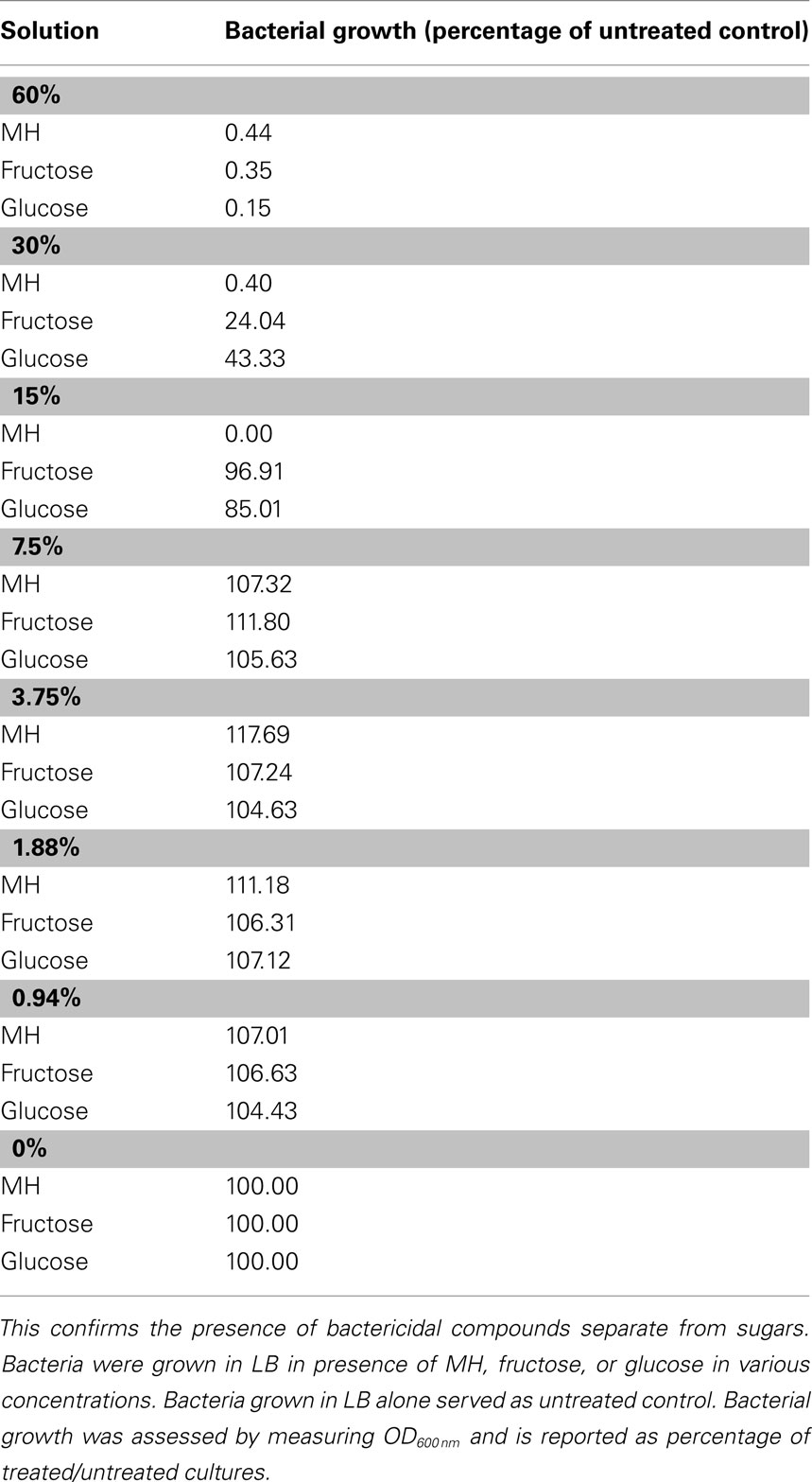
Table 1 . Manuka honey has a higher MIC than equivalent sugar solutions .
Certain honeys are known to be unusually effective against bacteria, a difference which is generally attributed to their nectar source ( Molan, 1992 ). We tested honeys on bacterial lawns and confirmed that MH has a significantly larger zone of inhibition than LH (data not shown). It is also known that heating honey reduces or destroys its bactericidal activity ( Molan, 1992 ), as shown in Figure 1 A. The difference between raw and heat-treated MH is clearly visible – raw honey produces a clear zone of 17 mm diameter, while heat limits killing to a small area in direct contact with the drop of honey (9 mm diameter). The decrease in bactericidal activity of heated honey was visible with both PA14 and pqsA isogenic mutant (Figure 1 A).
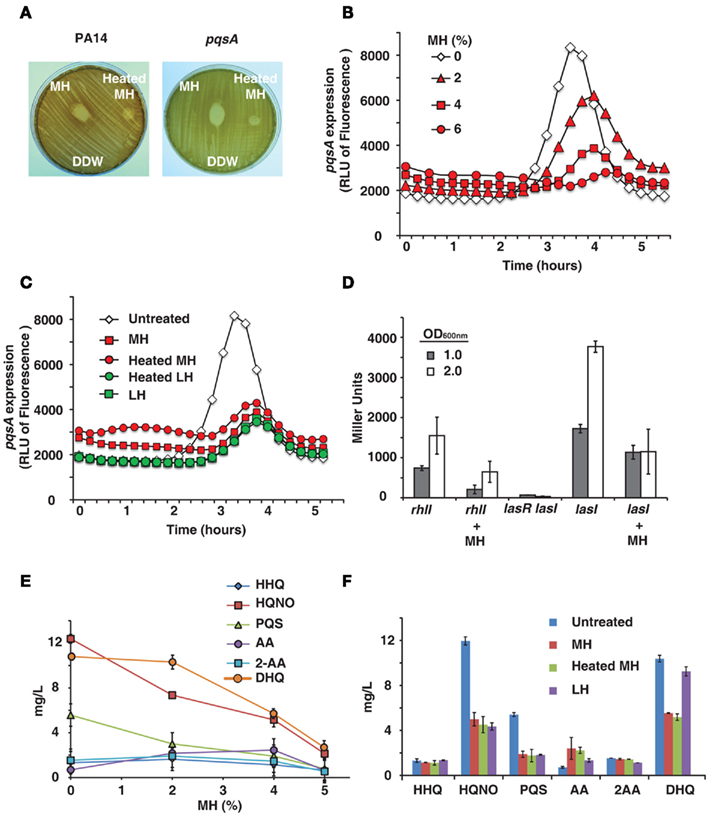
Figure 1. Honey inhibits expression of QS-related genes and molecules . (A) Shows manuka honey (MH) contains heat-sensitive bactericidal compounds. Raw MH, heat-treated MH and distilled water were dropped onto a P. aeruginosa lawn. Strains used were PA14 and a pqsA mutant. (B) Indicates honey contains a compound that reduces expression of pqsA . PA14 was grown in solutions of 2, 4, and 6% MH and pqsA expression was measured using a GFP (ASV) gene as a reporter. Fluorescence indicates pqsA expression, plotted here as a function of time. (C) Indicates inhibition of pqsA gene expression by honey is independent of honey source and unaffected by heat treatment. Experiment was performed as in (A) , with all honeys diluted to 4%. Samples used were MH and local honey (LH), with and without heat treatment. (D) The expression of lasI and rhlI genes in the presence of honey was measured using a Miller β-galactosidase assay with PA14 cells expressing lasI or rhlI driven by the lacZ promoter and a lasR<lasI double mutant cells as a negative control. Measurements were taken at OD 600 nm = 1.0 and 2.0 to determine gene expression at different cell densities. (E,F) Shows concentrations of MvfR low molecular weight QS-regulated molecules produced by the pqsABCD operon in the presence of honey. Samples used were MH and LH, with and without heat treatment.
Honey Inhibits QS Pathways and Production of QS-Regulated Small Molecules
Some of the most attractive non-lethal targets for an antimicrobial treatment are the QS networks, as inhibiting them would decrease bacterial virulence while possibly avoiding the selection pressure that conventional antibiotics exert ( Hentzer and Givskov, 2003 ). Therefore, we tested whether honey directly affects the expression of QS genes. We initially examined the activity of MvfR regulon via pqsA , since its expression is under direct MvfR control ( Déziel et al., 2005 ). To this end we used GFP (ASV) as a reporter of the pqsA gene expression ( Hazan et al., 2010 ).
PqsA expression was found to be significantly reduced by MH or LH, while cell growth was unaffected by up to 6% honey. Figure 1 B shows fluorescence over time – pqsA is expressed in the exponential stage of growth, and the graph shows a peak during this growth phase followed by a decline as the cells exit exponential growth. In addition to drastically reducing the height of this peak in the presence of honey, there was also a slight lag period likely caused by the bacteria adjusting to a new medium. A solution of 4% MH reduced pqsA gene expression by 50%, while not affecting final cell density.
The pqsA gene expression was also measured for different honeys at a fixed concentration of 4%, the highest concentration of honey that did not significantly impact final cell density. Figure 1 C shows fluorescence data for MH and LH, with and without heat treatment. No significant difference was observed between the two varieties of honey or between raw and heat-treated honey. A concentration of 4% of any honey sample had a similar impact on pqsA expression.
Honey’s impact on the las and rhl gene expression was quantified by using the β-galactosidase reporter. Figure 1 D shows a significant inhibition of both homoserine lactone synthase genes lasI and rhlI at two bacterial cell densities by 4% honey, especially prominent at OD 600 nm = 2.0.
As the pqsA gene is required for the synthesis of 4-hydroxy-2-alkylquinolines (HAQs) and activation of the MvfR regulon, the levels of the MvfR-regulated molecules, 4-hydroxy-2-heptylquinoline (HHQ), and its hydroxylated derivative 3,4-dihydroxy-2-heptylquinoline, also known as the Pseudomonas quinolone signal (PQS), as well as other more abundant MvfR-regulated low molecular weight molecules, 4-hydroxy-2-heptylquinoline N -oxide (HQNO), 2,4-dihydroxyquinoline (DHQ), anthranilic acid (AA), and 2-aminoacetophenone (2-AA) were assessed by LC–MS from PA14 cells grown in the presence of honey. Figures 1 E,F shows that increasing concentrations of honey led to an incremental reduction of the signal molecule levels, with a maximum reduction of approximately 50%. Most prominent reduction was observed with DHQ, HQNO, and PQS. Reduced levels of signal molecules and an accumulation of AA, a precursor molecule in the synthesis of HHQ, DHQ, HQNO, and 2-AA, are the most direct evidence of an impact on the MvfR regulon, as they are necessary for the feedback loop between MvfR and the genes it regulates. Reduction of DHQ, an abundant intermediate of HAQs further corroborates honey’s impact on MvfR regulon. Similarly, reduction of PQS and HQNO levels also suggests an impact on the LasR, system since HHQ is converted into PQS via the control of LasR, as is the last step of HQNO synthesis.
Observed inhibition of genes and signal molecules combined with impacts on downstream virulence factors in the pqs, las , and rhl operons shows a wide-reaching effect on QS networks.
Honey Impacts QS-Regulated Virulence Factors
Given honey’s impact on QS genes, our next step was to investigate its effect on virulence factors regulated by QS. In order to study this we examined virulence factors downstream to key QS genes in both the AHL and HAQ signal networks.
Pyocyanin is a toxic blue-green compound exclusive to P. aeruginosa , and is frequently measured as an index of the MvfR QS network that regulates it ( Rahme et al., 1997 ; Déziel et al., 2005 ; Hazan et al., 2010 ). Figure 2 A shows pyocyanin readings for P. aeruginosa grown in LB broth with 4% honey, and control with no honey. Pyocyanin production was reduced to 50% of the control by both MH and LH. Moreover, Figure 2 A also shows that heat-treated honey equally inhibits pyocyanin production despite lacking bactericidal properties. Inhibition of pyocyanin was also observed in minimal medium (M9; data not shown).
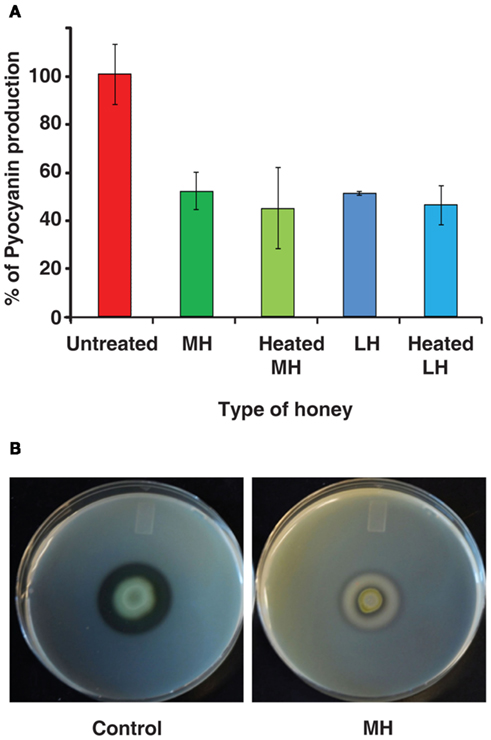
Figure 2. Honey impacts production of virulence factors . (A) Indicates honey decreases the production of pyocyanin, a virulence factor regulated by the MvfR QS network of P. aeruginosa . Pyocyanin is displayed as a percentage normalized to the control (no honey added). (B) demonstrates the effect on extracellular protease production by honey. Milk plates were inoculated with 2 μL of bacterial culture and incubated for 48 h at 24°C.
In addition, we found that honey also inhibits production of extracellular proteases, which are known to be under the control of MvfR and the las operon – part of the AHL network ( Déziel et al., 2004 ). When P. aeruginosa was grown on skim milk plates, the addition of honey to the plates almost completely eliminated the clear halo around colonies caused by breakdown of casein. Control plates exhibited a 7-mm halo, while colonies on honey plates only created a 2-mm halo (Figure 2 B).
Impact on two separate QS-regulated virulence factors suggests that honey affects the expression of the genes that regulate them. This would indicate an underlying interaction between honey and the QS networks of P. aeruginosa .
Quorum Sensing Inhibition by Honey is Associated with Sugar Content
Our results have shown that the components of honey responsible for QS inhibition are likely: (a) present in all types of honey regardless of nectar source, and (b) unaffected by heat treatment. The most obvious candidate is sugar, which is the largest component in all types of honey. To examine the role of honey’s sugar component, we substituted sugar solutions for honey in tests of QS-related behaviors.
A pqsA /GFP assay carried out with sugar solutions in LB broth recreated honey’s inhibitory effect almost exactly. As honey is roughly 80% sugar, the solutions used were compared to equivalent honey dilutions. Figure 3 A shows that sugar solutions decreased pqsA gene expression and that glucose was the most potent of the ones tested. A concentration of only 1% glucose decreased pqsA gene expression by about 50% similarly to MH or LH (Figure 3 A).
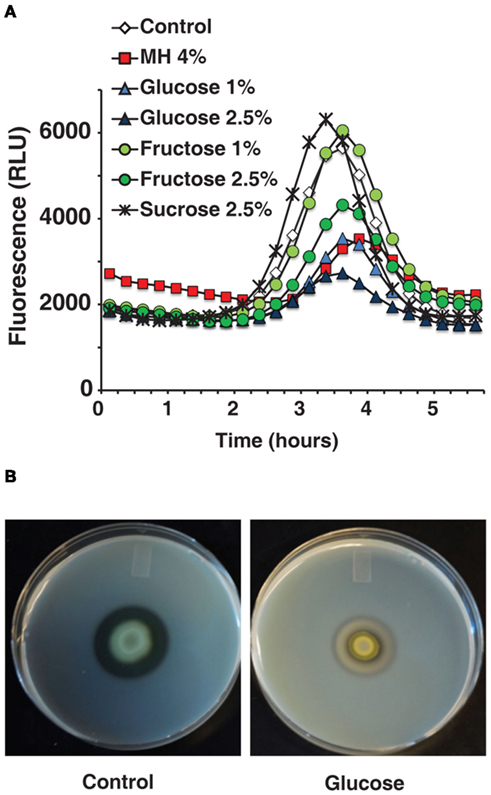
Figure 3. The effects of honey on QS-related genes and virulence factors can be reproduced by comparable sugar solutions . (A) Indicates that the inhibitory effect of diluted honey on pqsA can be reproduced using sugar solutions. (B) Shows the effect on extracellular proteases caused by 2.5% glucose in milk plates.
Figure 3 B shows that 2.5% glucose added to a milk plate inhibited production of extracellular proteases in a similar manner to 4% MH, again almost eliminating the clear halo around PA14 cells. Additionally, in a pyocyanin assay in M9 minimal medium the addition of 1% glucose inhibited pyocyanin almost completely with no effect on growth (data not shown). The low concentration of glucose and the simple nature of the medium rule out osmotic stress or related effects as a possible cause.
The ability to replicate the impact caused by honey in multiple tests, both on gene expression and on QS-related virulence factors, supports the hypothesis that sugar is in active component in QS inhibition.
This study characterizes honey’s interactions with the QS systems of P. aeruginosa , using sub-inhibitory concentrations (6% honey and below) to systematically examine the impact of honey on living bacterial cells. Our novel approach differs significantly from the use of high concentrations of honey to study bactericidal effects, and reveals another layer of information regarding honey’s unusual ability to fight infections.
Firstly, honey causes a significant reduction in expression of both P. aeruginosa QS pathways. Inhibition of pqsA reveals an impact on the synthesis of the HAQ signaling molecules, PQS and HHQ, which are required for the activity of MvfR and full expression of the MvfR-regulated virulence factors such as pyocyanin – a finding further reinforced by the accumulation of AA observed, as it is used as a precursor in the synthesis of these molecules (Figure 1 E). lasI and rhlI , however, are part of the lasR and rhlR systems that make up the homoserine lactone network ( Hazan et al., 2010 ). The rhlR system is linked to MvfR, in addition to regulating another array of virulence factors such as protease production, motility, and biofilm formation ( Caiazza et al., 2007 ; Patriquin et al., 2008 ). However, the connection between the LasR and MvfR systems, in reference to honey, means that further research is needed to determine whether honey’s inhibition of the MvfR regulon is a direct impact or a secondary effect through the LasR system.
More directly, honey’s negative impact on virulence factors regulated by these networks serves to confirm our hypothesis. Reduced production of pyocyanin is consistent with the observed inhibition of the hydroxyquinoline-based MvfR system, which controls its production. Honey also inhibited expression of extracellular proteases, which are regulated by the las operon ( Déziel et al., 2004 ). This operon is known to be regulated both by MvfR and by lasR QS systems. Honey’s impact on bacterial behaviors associated with QS combined with its inhibition of key genes in both known signaling networks strongly suggests that it has a wide-reaching effect on QS and associated virulence factors.
Comparisons between the nature of honey’s bactericidal components and those inhibiting QS show that there are two separate mechanisms at work. Previous studies show that bactericidal activity is likely caused by enzymes or proteins. Our results show that honey’s previously unknown ability to inhibit QS is at least partially caused by honey’s sugar content. Impacts on pqsA , pyocyanin, and extracellular proteases were all reproducible by comparable glucose or fructose solutions. Sugar content, mainly glucose and fructose, is constant regardless of nectar source because honeybees judge the ripeness of honey by sugar concentration. It is not destroyed by heat, which matches the characteristics of the component inhibiting QS.
The above results provide valuable insights on the medicinal use of honey. Consistent with previous studies, the nectar source is important to honey’s bactericidal properties, with MH generally acknowledged as one of the most active. In addition to being a potent treatment in its own right, honey’s ability to disrupt QS explains the synergistic effect seen when honey is combined with antibiotics, as interfering with cell-to-cell communication prevents bacteria from acting as a multicellular entity and makes them more susceptible to attack. QS-related behaviors are one of the most dangerous aspects of a bacterial infection – QS is key in activating multiple virulence factors, forming highly resilient biofilms and even encouraging the formation of antibiotic tolerant cells. Therefore, inhibiting QS provides a new and little-explored tactic against bacteria: the ability to weaken an infection and disrupt virulence, potentially without exerting selection pressure for resistance.
The lingering question is the exact mechanism of QS inhibition by honey’s sugar content. The concentrations of sugar used did not inhibit bacterial growth at all, indicating that the effect is not related to osmotic stress. As QS controls responses to environmental stimuli such as nutrition, one possible explanation is that the increased sugar causes a change in the metabolic pathways used by the bacteria in such a way that QS genes are not activated or are inhibited. It is possible that the presence of an easy food source in the form of sugar leads to less stress on the cells and a corresponding lack of aggression. This might also explain the difference in effect between the two sugars added – perhaps different genes are being activated to metabolize each. Additional experiments are needed to determine whether the effect is limited to simple sugars like glucose and fructose or whether other sugars or compounds could produce similar results. Future studies will be needed to address: (1) how sugar impacts QS-related behavior; and (2) whether there are other elements in honey that catalyze or enhance sugar’s effect.
In summary, honey’s ability to counter bacterial infections arises from two main factors: bactericidal compounds and QS inhibition. As honey is a highly complex substance there may be further contributing factors or interdependent components, necessitating further research, including possible metabolic changes that may occur as a result of sugars utilization. In the meantime it stands out as a promising rediscovered treatment whose strength lies in its ability to counter bacterial virulence on multiple levels. Honey itself may prove to be one new weapon in the battle against antibiotic resistance, but understanding and replicating its attack on bacteria by multiple mechanisms could be the key to an entirely new strategy to counter infections.
Conflict of Interest Statement
The authors declare that the research was conducted in the absence of any commercial or financial relationships that could be construed as a potential conflict of interest.
Abd-El Aal, A. M., El-Hadidy, M. R., El-Mashad, N. B., and El-Sebaie, A. H. (2007). Antimicrobial effect of bee honey in comparison to antibiotics on organisms isolated from infected burns. Ann. Burns Fire Disasters 20, 83–88.
Pubmed Abstract | Pubmed Full Text
Ahern, T. J., and Klibanov, A. M. (1985). The mechanisms of irreversible enzyme inactivation at 100C. Science 228, 1280–1284.
Pubmed Abstract | Pubmed Full Text | CrossRef Full Text
Alandejani, T., Marsan, J., Ferris, W., Slinger, R., and Chan, F. (2009). Effectiveness of honey on Staphylococcus aureus and Pseudomonas aeruginosa biofilms. Otolaryngol. Head Neck Surg. 141, 114–118.
Aloush, V., Navon-Venezia, S., Seigman-Igra, Y., Cabili, S., and Carmeli, Y. (2005). Multidrug-resistant Pseudomonas aeruginosa : risk factors and clinical impact. Antimicrob. Agents Chemother. 50, 43–48.
CrossRef Full Text
Arias, A. A., and Murray, B. E. (2009). Antibiotic-resistant bugs in the 21st century – a clinical super-challenge. N. Engl. J. Med. 360, 439–443.
Atkinson, S., and Williams, P. (2009). Quorum sensing and social networking in the microbial world. J. R. Soc. Interface 6, 959–978.
Bassler, B. L. (1999). How bacteria talk to each other: regulation of gene expression by quorum sensing. Curr. Opin. Microbiol. 2, 582–587.
Caiazza, H. C., Merritt, J. H., Brothers, K. M., and O’Toole, G. A. (2007). Inverse regulation of biofilm formation and swarming motility by Pseudomonas aeruginosa PA14. J. Bacteriol. 189, 3603–3612.
Cao, H., Krishnan, G., Goumnerov, B., Tsongalis, J., Tompkins, R., and Rahme, L. G. (2001). A quorum sensing-associated virulence gene of Pseudomonas aeruginosa encodes a LysR-like transcription regulator with a unique self-regulatory mechanism. Proc. Natl. Acad. Sci. U.S.A. 98, 14613.
Cornelis, P. (ed.). (2008). Pseudomonas: Genomics and Molecular Biology . Norfolk: Caister Academic Press.
Crane, E. (ed.). (1975). Honey: A Comprehensive Survey . London: Heinemann.
Déziel, E., Gopalan, S., Tampakaki, A. P., Lépine, F., Padfield, K. E., Saucier, M., Xiao, G., and Rahme, L. G. (2005). The contribution of MvfR to Pseudomonas aeruginosa pathogenesis and quorum sensing circuitry regulation: multiple quorum sensing-regulated genes are modulated without affecting lasRI, rhlRI or the production of N-acyl-L-homoserine lactones. Mol. Microbiol. 55, 998–1014.
Déziel, E., Lépine, F., Milot, S., He, J., Mindrinos, M. N., Tompkins, R. G., and Rahme, L. G. (2004). Analysis of Pseudomonas aeruginosa 4-hydroxy-2-alkyquinolines (HAQs) reveals a role for 4-hydroxy-2-heptylquinoline in the cell- to-cell communication. Proc. Natl. Acad. Sci. U.S.A. 101, 1339–1344.
Efem, S. E. E. (1988). Clinical observations on the wound-healing properties of honey. Br. J. Surg. 75, 679–681.
Efem, S. E. E., and Iwara, C. I. (1992). The antimicrobial spectrum of honey and its clinical significance. Infection 20, 227–229.
Freire-Moran, L., Aronsson, B., Manz, C., Gyssens, I. C., So, A. D., Monnet, D. L., and Cars, O. (2011). Critical shortage of new antibiotics in development against multidrug resistant bacteria – time to react is now. Drug Resist. Updat. 14, 118–124.
Fuqua, C., Parsek, M. R., and Greenberg, E. P. (2001). Regulation of gene expression by cell-to-cell communication: acyl-homoserine lactone quorum sensing. Annu. Rev. Genet. 35, 439–468.
Gallagher, L. A., McKnight, S. L., Kuznetsova, M. S., Pesci, E. C., and Manoil, C. (2002). Functions required for extracellular quinolone signaling by Pseudomonas aeruginosa . J. Bacteriol. 184, 6472–6480.
Hazan, R., He, J., Xiao, G., Dekimpe, V., Apidianakis, Y., Lesic, B., Astrakas, C., Déziel, E., Lépine, F., and Rahme, L. G. (2010). Homeostatic interplay between bacterial cell–cell signaling and iron in virulence. PLoS Pathog. 6, e1000810. doi:10.1371/journal.ppat.1000810
Hentzer, M., and Givskov, M. (2003). Pharmacological inhibition of quorum sensing for the treatment of chronic bacterial infections. J. Clin. Invest. 112, 1300–1307.
Jeffrey, A. E., and Echazarretta, C. M. (1996). Medical uses of honey. Rev. Biomed. 7, 43–49.
Kwakman, P. H. S., te Velde, A. A., de Boer, L., Speijer, D., Vandenbroucke-Grauls, C., and Zaat, S. (2010). How honey kills bacteria. FASEB J. 24, 2576–2582.
Kwakman, P. H. S., Van den Akker, J. P. C., Gûçlû, A., Aslami, H., Binnekade, J. M., de Boer, L., Boszhard, L., Paulus, F., Middelhoek, P., te Velde, A. A., Vandebroucke-Grauls, C. M. J. E., Schultz, M. J., and Zaat, S. A. J. (2008). Medical-grade honey kills antibiotic-resistant bacteria in vitro and eradicates skin colonization. Clin. Infect. Dis. 46, 1677–1682.
Lépine, F., Déziel, E., Milot, S., and Rahme, L. G. (2003). A stable isotope dilution assay for the quantification of the Pseudomonas quinolone signal in Pseudomonas aeruginosa cultures. Biochim. Biophys. Acta 1622, 36–41.
Miller, J. H. (1972). “Assay of β-galactosidase” in Experiments in Molecular Genetics , ed. J. H. Miller (Cold Spring Harbor, NY: Cold Spring Harbor Laboratory), 352–355.
Molan, P. C. (1992). The antibacterial activity of honey: 2. Variation in the potency of antibacterial activity. Bee World 73, 59–76.
Molan, P. C. (1999). The role of honey in the management of wounds. J. Wound Care 8, 415–418.
Patriquin, G. M., Banin, E., Gilmour, C., Tuchman, R., Greenberg, E. P., and Poole, K. (2008). Influence of quorum sensing and iron on twitching motility and biofilm formation in Pseudomonas aeruginosa . J. Bacteriol. 190, 662–671.
Rahme, L. G., Stevens, E. J., Wolfort, S. F., Shao, J., Tompkins, R. G., and Ausubel, F. M. (1995). Common virulence factors for bacterial pathogenicity in plants and animals. Science 268, 1899–1902.
Rahme, L. G., Tan, M.-W., Le, L., Wong, S. M., Tompkins, R. G., Calderwood, S. B., and Ausubel, F. M. (1997). Use of model plants hosts to identify Pseudomonas aeruginosa virulence factors. Proc. Natl. Acad. Sci. U.S.A. 94, 13245–13250.
Shiner, E. K., Rumbaugh, K. P., and Williams, S. C. (2005). Inter-kingdom signaling: deciphering the language of acyl homoserine lactones. FEMS Microbiol. Rev. 29; 935–947.
So, A. D., Gupta, N., and Cars, O. (2010). Tackling antibiotic resistance. BMJ 340, c2071.
Sokol, P. A., Ohman, D. E., and Iglewski, H. B. (1979). A more sensitive plate assay for detection of protease production by Pseudomonas aeruginosa . J. Clin. Microbiol. 9, 538–540.
Tomasz, A. (1994). Multiple-antibiotic-resistant pathogenic bacteria – a report on the Rockefeller University Workshop. N. Engl. J. Med. 330, 1247–1251.
Wade, D. S., Calfee, M. W., Rocha, E. R., Ling, E. A., Engstrom, E., Coleman, J. P., and Pesci, E. C. (2005). Regulation of Pseudomonas quinolone signal synthesis in Pseudomonas aeruginosa . J. Bacteriol. 187, 4372–4380.
White, J. W., Reithof, M. L., Subers, M. H., and Kushnir, I. (1962). Composition of American Honey. US Dept. Agric. Tech. Bull. 1261, 1–124.
Wijesinghe, M., Weatherall, M., Perrin, K., and Beasley, R. (2009). Honey in the treatment of burns: a systematic review and meta-analysis of its efficacy. N. Z. Med. J. 122, 47–60.
Willix, D. J., Molan, P. C., and Haroot, C. G. (2008). A comparison of the sensitivity of wound-infecting species of bacteria to the antibacterial activity of manuka honey and other honey. J. Appl. Microbiol. 73, 388–394.
Yang, L., Barken, K. B., Skindersoe, M. E., Christensen, A. B., Givskov, M., and Tolker-Nielsen, T. (2007). Effects of iron on DNA release and biofilm development by Pseudomonas aeruginosa . Microbiology 153, 1318–1328.
Zumla, A., and Luat, A. (1989). Honey – a remedy rediscovered. J. R. Soc. Med. 82, 384–385.
Keywords: honey, Pseudomonas , quorum-sensing, infection, anti-infective, QS inhibition, mvfR(pqsR) , sugars
Citation: Wang R, Starkey M, Hazan R and Rahme LG (2012) Honey’s ability to counter bacterial infections arises from both bactericidal compounds and QS inhibition. Front. Microbio. 3 :144. doi: 10.3389/fmicb.2012.00144
Received: 19 January 2012; Paper pending published: 09 February 2012; Accepted: 26 March 2012; Published online: 11 April 2012.
Reviewed by:
Copyright: © 2012 Wang, Starkey, Hazan and Rahme. This is an open-access article distributed under the terms of the Creative Commons Attribution Non Commercial License , which permits non-commercial use, distribution, and reproduction in other forums, provided the original authors and source are credited.
*Correspondence: Laurence G. Rahme, Molecular Surgical Laboratory, Department of Surgery, Harvard Medical School and Massachusetts General Hospital, 340 Thier Research Building, 50 Blossom Street, Boston, MA 02114, USA. e-mail: rahme@molbio.mgh.harvard.edu
† Present address: Melissa Starkey, American College of Physicians, Philadelphia, PA, USA
Disclaimer: All claims expressed in this article are solely those of the authors and do not necessarily represent those of their affiliated organizations, or those of the publisher, the editors and the reviewers. Any product that may be evaluated in this article or claim that may be made by its manufacturer is not guaranteed or endorsed by the publisher.

IMAGES
COMMENTS
May 30, 2008 · Honey is antibacterial and can prevent growth of most types of bacteria. This experiment compares the effect of different types of honey on bacteria growing on agar plates. Purpose. To compare the antibacterial effect of different honey types. Keywords. Honey, antibacterial, bacteria, agar, experiment.
Currently, many researchers have reported the antibacterial activity of honey and found that natural unheated honey has some broad-spectrum antibacterial activity when tested against pathogenic bacteria, oral bacteria as well as food spoilage bacteria,. In most ancient cultures honey has been used for both nutritional and medical purposes.
2.6.1. Antibacterial effect of honey. The antibacterial efficacy of honey was initially recognized in 1892; however, in modern medicine, it is used only to a limited extent due to the absence of scientific support (Mohapatra et al. 2011). Honey exhibits antibacterial activity against numerous bacteria in different environments.
Honey is known to possess antimicrobial activity and has been used to cure many skin infections.11,12,13,18,19 In our experiment, we observed that all the honey samples examined possessed antibacterial activity against H. pylori with the natural Black Forest honey from the Black Forest in Germany showing the highest antibacterial activity. This ...
Sep 1, 2017 · For all tested honey types, there was total inhibition of bacterial growth in both MSSA and MRSA at the highest concentration of 50% (v/v) (Table 2).At a concentration of 10% (v/v), all three tested Manuka Honeys produced a significant decline in both MSSA and MRSA; N. sativa and Sidr honey did not cause a significant decrease in bacterial growth (Table 2).
Apr 1, 2021 · Various parameters affect the antibacterial potential of honey including its low water content (low water activity), high viscosity, acidity, and H 2 O 2 content. In addition, various compounds are associated with honey and provide its antibacterial potential, including phytochemicals, peptides, nonperoxidase glycopeptides, and proteins that are notable features of honey and are associated ...
Jun 19, 2018 · Throughout the experiments, the agar-well diffusion method was applied and used with a 75% solution of honey, for practical reasons, to determine the antibacterial effects of the honey samples. The 75% solution of honey had significant effect on the bacterial growth, while no effect was seen for the 20% solution of honey.
Apr 10, 2012 · By diluting honey to sub-inhibitory levels, we were able to study its impact on bacterial coordinated behavior, and discovered that honey inhibits bacterial quorum sensing (QS). Experiments to characterize and quantify honey’s effect on the QS networks of Pseudomonas aeruginosa revealed that low concentrations of honey inhibited the ...
Mar 7, 2011 · The combination of honey as a natural antibacterial agent, refractory to antibiotic-resistant bacteria, with dimexidum leads to suppression of growth in vitro most of the tested pathogens.
Apr 3, 2019 · The antibacterial activity of honey was first recognized in 1892; however, it has a limited use in modern medicine due to lack of scientific support . Honey is the nectar collected from flowers by bees. It contains 15% to 20% water and 80% to 85% sugar.